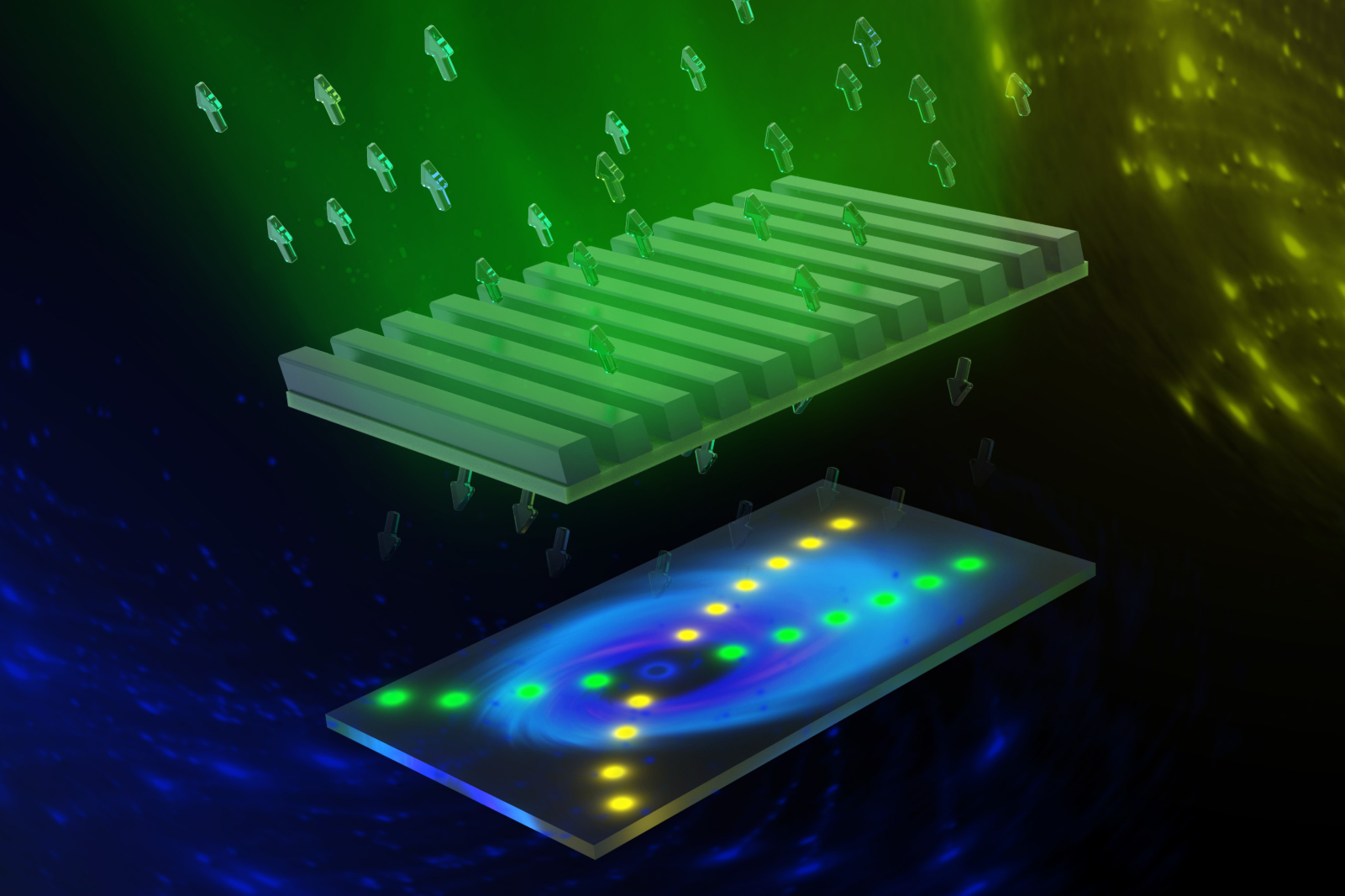
A new study published in Nature describes a novel design of optical devices that radiate light in a single direction. This new single-sided radiation channel for light can be used in a wide array of optoelectronic applications to reduce energy consumption in optical fiber networks and data centers, demonstrating how new theories from the field of topology can be used to improve existing technologies. The research was conducted by Ph.D. student Jicheng Jin, assistant professor Bo Zhen, both of Penn, and researchers at Peking University and the Massachusetts Institute of Technology.
Why is single-sided radiation so important in optical fiber networks? Light tends to flow in optical fibers in a single direction, like water flows through a pipe. For light, on-chip couplers connect fibers to chips, where light signals are generated, amplified, or detected. While most light going through the coupler continues through to the fiber, some of the light travels in the opposite direction, so the couplers act as “leaky” pipes.
A large portion of energy consumption in data traffic is due to this radiation loss, and, with data center energy consumption equivalent to 2% of global demand, researchers are highly motivated to reduce this type of energy loss. However, despite tremendous progress in this field, previous studies consistently reported a minimum loss of 25% at each interface between optical fibers and chips. Data centers require complex and interwoven systems of nodes, so this loss quickly multiplies as light travels through a convoluted network.
“Sometimes you may need to pass five interfaces, and loss cascades to 80% if you use existing devices. In fact, extra energy and elements are needed to amplify and relay the signal again and again, which introduces noise, lowers signal-to-noise ratio, and, ultimately, reduces communication bandwidth,” says Jin.
“We found the 25%-loss lower bound to be very strange,” says Zhen about this consistently reported level of loss.
After studying the system in more detail, the researchers discovered that breaking left-right symmetry in their device reduced this loss to zero. “The conclusion was a little unexpected. Since we want the coupler to radiate towards the top rather than the bottom, it seems natural to break up-down symmetry, like the shape on the bottom left. However, it turns out we must also make it left-right asymmetric to completely suppress the bottom radiation, like the one on the bottom right,” says Jin.
To better understand this phenomenon, the researchers developed a theory based on topological charges, which forbid radiation in a specific direction. For a coupler with both up-down and left-right symmetries, there is one charge on each side forbidding the radiation in the vertical direction.
“Imagine it as two-part glue. By breaking the left-right symmetry, the topological charge is split into two half charges, and the two-part glue is separated so each part can flow. By breaking the up-down symmetry, each part flows differently on the top and the bottom, so the two-part glue combines only on the bottom, eliminating radiation in that direction. It’s like a leaky pipe has been fixed with a topological two-part glue,” says Zhen.
The team eventually settled on a design with a series of slanted bars, which break left-right and up-down symmetries at the same time. To fabricate these structures, the researchers developed a novel etching method, with silicon chips placed on a wedge-like substrate. This allows etching to occur at a slanted angle, whereas standard etchers can only create vertical side walls. After fabricating the device, the researchers were able to confirm the low energy loss that was predicted by their theory.
“Experimentally, we found that radiation towards the bottom is 500 times weaker than radiation towards the top in our device, minimizing energy loss,” says Jin. “In addition, our design is very robust to fabrication errors. One limiting factor in our current design is the bandwidth. Right now, we can cover about 26nm, which is decent but not optimal, and our next step is to further improve this to 200nm.”
“These exciting results have the potential to spur new research investments for Army systems,” says Michael Gerhold of the Army Research Laboratory. “Not only do the coupling efficiency advances have potential to improve data communications for commercial data centers, but the results carry huge impact for photonic systems where much lower intensity signals can be used for the same precision computation, making battery powered photonic computers possible.”
This current system is practical due to its simplicity in fabrication and design. In the future, the team hopes to further develop this etching technique to be compatible with existing industrial processes, and the researchers expect applications that can both help light travel more efficiently at short distances, such as between an optical fiber cable and a chip in a server, and over longer distances, such as long-range Lidar systems. “This method is relatively clear and straightforward, so I think everyone can easily get this reliable structure,” says Jin.
This research was supported by the U.S. Army Research Office, AFRL, the MIT Lincoln Laboratory, the Natural Science Foundation of China, and HPCP of Peking University.