Liquid Crystal Turns Water Droplets Into ‘Gemstones,’ Penn Research Shows
Liquid crystals are remarkable materials that combine the optical properties of crystalline solids with the flow properties of liquids, characteristics that come together to enable the displays found in most computer monitors, televisions and smartphones.
In a study published in the Proceedings of the National Academy of Sciences, researchers from the University of Pennsylvania and Swarthmore College describe new research into a type of liquid crystal that dissolves in water rather than avoids it as do the oily liquid crystals found in displays. This property means that these liquid crystals hold potential for biomedical applications, where their changing internal patterns could signal the presence of specific proteins or other biological macromolecules.
The researchers placed these liquid crystals into water droplets, which in turn were placed in oil, producing an emulsion. At high enough concentrations within the droplets, the liquid crystals exhibit a twisting pattern visible under an optical microscope.
At even higher concentrations, however, the liquid crystals display an even more unusual behavior: their constituent molecules stack to form columns that organize into crystal-like structures and transform the normally spherical water droplets into faceted fluid gemstones.
The research was led by postdoctoral fellow Joonwoo Jeong and graduate student Zoey Davidson, both members of Arjun Yodh’s research group at Penn. Yodh directs the Laboratory for Research on the Structure of Matter and is a professor in the Department of Physics and Astronomy in the School of Arts and Sciences. Rounding out the collaboration are Tom Lubensky, also a professor in Penn’s Department of Physics and Astronomy, and Peter Collings, a professor in the Department of Physics and Astronomy at Swarthmore.
“Our long range goal,” Yodh said, “is to gain more understanding and control over this liquid crystal system in order to harness its abilities to make useful new materials. Research with this type of liquid crystal is at a stage similar to that of display liquid crystals in the early ‘70s. The first displays were limited until their properties were really understood.”
The type of liquid crystals the researchers investigated in this study are lyotropic chromonic liquid crystals, or LCLCs. They contain organic salts commonly known as “Sunset Yellow” or “Yellow 6,” a widely used food dye found in orange soda, cheese-flavored snacks and many other products.
When placed in water, individual molecules of the dye stack up like poker chips, forming microscopic rods. Then the liquid-crystal containing water drops are mixed into an oil suspension along with soap-like surfactants. With one end attracted to water and the other end repelled by it, the surfactant molecules travel to the surface of the drop once it is immersed in oil. This helps stabilize the drop into a sphere, which is the optimal shape for minimizing surface energy.
Once the droplets are in the oil emulsion, the rods interact with one another to form different kinds of aligned phases. Unlike the liquid crystals found in displays, the phases or patterns formed by lyotropic liquid crystals are dependent on their concentration. To vary LCLC concentration, the researchers started with different concentrations of the dye molecules in the droplets and permitted the concentration to increase further as water diffused out of the droplets.
At a sufficiently high level of concentration, the rods are pushed close enough together that they begin to align parallel to one another, forming what is known as a “nematic” phase. This gives the droplet a well-defined north-south axis running through its center. Rods near this axis line up in parallel, until they get to the two “poles” where the rods are not oriented with respect to one another.
This pattern results from the liquid crystal’s tendency to rearrange itself into the “easiest,” lowest-energy state. And, like the liquid crystals found in displays, this state can be influenced by external effects, and the resulting behavior may be harnessed for applications.
“A lot of the work that is done to understand these types of liquid crystal systems,” Collings said, “is to put two competing influences on them, such as the molecules’ natural tendency to line up perfectly versus some confinement or external field that says, ‘We're not going to let you do that.’”
The confinement in this case is the spherical surface of the drop.
“The rods want to line up parallel to each other,” Jeong said, “but they also want to line up with the boundary of the drop, and they can’t do both at once. Thus the rods must undergo deformation. Interestingly, in this case, they twist to accommodate this confinement.”
This twist does not change the shape of individual rods, which remain straight, but rather changes the rods’ orientation relative to each other. Looking at the droplet so that the rods are pointed vertically along its axis, this twist occurs along lines from the axis to the droplet’s surface. Like clothespins clipped to those lines, individual rods can rotate out of their original vertical position, twisting an average of more than 90 degrees.
Most oil-based liquid crystals found in displays respond to this kind of confinement similarly in that they form a central axis with two poles, but differently in that they don’t twist away from that axis.
“This means that, unlike with display liquid crystals, anything we do with these LCLCs must take this twist into account,” Collings said.
These patterns depend on the drop’s surface properties, so the twisting of the rods could be influenced by outside molecules that attach to the surface and change its boundary conditions.
“This property of the LCLCs affords them with potential to be a detector,” Yodh said. “Small variations at the molecular level could induce changes in the liquid crystal structure that are viewable by microscopy.”
As the concentration of the drops increases further, however, the liquid crystal evolves from the nematic phase to the “columnar” phase, in which the rods pack together like bundles of straws. This packing prevents the rods from twisting, so they respond to the cue from the drop’s surface by lining up into arrays of columns, keeping parallel to the boundary and circling the drop’s central axis.
Once in the columnar phase, the ordered internal structure of the liquid crystal creates an environment such that a sphere is no longer the optimal shape for minimizing the drop’s surface energy. The surface is pulled in towards the ordered collection of rods, and the exterior of the drop begins to conform to the hexagonal packing pattern of the columns, forming facets.
“As far as we know, these are the first emulsion drops to contain liquid crystals in the columnar phase,” Yodh said. “It is amazing to see the surface of the drop evolve. I have never seen a drop of water shaped like a gemstone!”
The research was supported by the National Science Foundation and Penn’s Materials Research Science and Engineering Center.
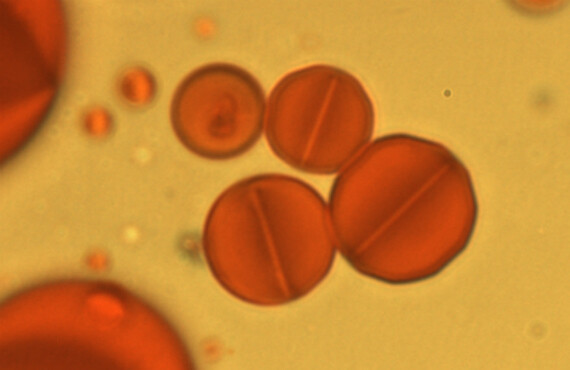