Penn Chemists Discover a Fluorophore That Will Open New Doors in Optical Imaging
Researchers at the University of Pennsylvania have discovered a new, smaller type of fluorophore derived from the amino acid tryptophan, commonly found in turkey, that will enable new spectroscopic and microscopic measurements of proteins, opening new doors in the study of biological processes.
Fluorophores, chemical compounds that emit photons when excited, are key to fluorescent microscopy, a technique that takes advantage of this light to allow a wide range of biochemical and biophysical processes and interactions to be studied at various length scales.
“In biology, seeing is believing,” said Mary Rose Hilaire, who completed her Ph.D. in physical chemistry at Penn in May. “We want to be able to biologically image cells or proteins while they're still alive and carrying out their function. Fluorescence microscopy is not only compatible with living cells, meaning the cells can still be alive while scientists are taking the measurements; it's essentially background free, so whatever you put in that fluoresces, that's what you're looking at. You don't have to worry about getting signals from other things in the system.”
Feng Gai, the Edmund J. and Louise W. Kahn Endowed Term Professor of Chemistry in the School of Arts & Sciences, compared the mechanism that makes this type of imaging possible to glow sticks.
“If you break them up in the dark you see color,” said Gai. “That's based upon this principle. You have photons coming out of a dye, making the stick glow.”
Because of the size of proteins and of cells, fluorescence microscopy affords scientists the spectral and spatial resolution needed to get the details about the system being studying. But until now, in applications where a small fluorescence reporter is required or desirable, the choice of fluorophores has been rather limited.
The research was published in the Proceedings of the National Academy of Sciences and was led by graduate students Hilaire and Ismail Ahmed under the guidance of Gai in collaboration with William F. DeGrado, a professor of pharmaceutical chemistry at University of California, San Francisco.
Before this research, there were only two classes of common fluorophores: organic dyes and fluorescent proteins like green fluorescent protein, or GFP. While there are unnatural amino acid-based fluorophores, none is as close in size to canonical amino acids as the one described in this study. Moreover, this fluorophore emits light in the visible region of the spectrum and is also extremely bright, which is hallmark of a good fluorophore. This means that, when one shines light on them, they absorb a lot of that light and then release most of that energy through fluorescence.
Scientists can use these fluorophores in a way similar to a GPS tracker, Ahmed said, to follow a specific protein in a cell over time and see where it goes. They can also harness the power of genetics and chemistry to label different proteins with different color fluorophores and see if they’re interacting with each other. A third way they’re used is as a reference point to make maps of cells to know where things are at any given time to study a myriad of things such as changes in cancer cells versus normal cells.
“It's become a hallmark in biological research,” Ahmed said, “It's been used for almost infinite applications.”
However, both GFPs and organic dyes have disadvantages. For organic dyes, scientists have to incorporate them into cells; they can't use any of the genetic material in a cell and get it to produce that organic dye.
While GFPs can be expressed in cells to fluorescently label proteins, GFP itself is rather large. So, if scientists are tagging it to another protein, they don't know what it's doing to the protein that they’re trying to look at.
The formation of GFP is also relatively slow and needs several hours to produce its color, which doesn’t lend itself well to following fast biological processes such as protein folding.
“In the two to four hours it takes for the color to form,” Gai said, “you’re still in the dark.”
The researchers wanted to be able to have a fluorophore that had a very high brightness in the visible region that was on the size scale of an amino acid. This would enable it to be incorporated into a protein and not perturb any of the physical properties of the system.
There are 20 naturally occurring amino acids that make up proteins, but only three of them, phenylalanine, tyrosine and tryptophan, are fluorescent. However, these amino acids don’t absorb enough light or give off enough photons in the form of fluorescence for use in biological imaging. They also all absorb and emit in the UV region, which doesn’t generate visible light and would damage the cells being studied.
“We want to be able to somehow add something to these amino acids,” Hilaire said, “to try to tune and change those photophysical properties so that they're more advantageous to study proteins in cells.”
Hilaire had been working on a different experiment when she noticed that the tryptophan derivative fluoresced when a nitrile group was appended to the 4 position of the ring, which is basically a carbon and nitrogen atom arranged in a triple bond attached to the tryptophan ring structure.
“I told them it must be a mistake,” Gai said. “It was too good to be true. We are always on the lookout for better fluorophores, but this is just one of those breakthroughs that couldn’t be predicted.”
The researchers decided to do a proof-of-concept experiment, taking an antimicrobial peptide and putting their amino acid on the end of it. The purpose of the experiment was to label the cells to show that they could see the process of the peptide binding to and degrading the membrane through different mechanisms.
For their experiment the researchers used a lamp-based microscope. After they appended the unnatural amino acid to the molecule, they watched it turn blue in the microscope and illuminate the cell, which it wouldn’t do under normal conditions.
“Almost all the light that you shine comes back through fluorescence,” Hilaire said, “which is perfect for biological imaging because that means you have a lot of signal, and it also means you don't have to use that much, so then you don't have to perturb your system too much because you can have a low concentration of your fluorophore.”
The amino acid also has a great photostability, which means that, unlike other fluorophores, it maintains its intensity for a relatively long time.
The researchers believe that the fact that they were able to get decent images using such low concentrations of the amino acid and just an LED, which is significantly less powerful than the lasers that would typically be used, is a testament to the potential of this technique in biological spectroscopy.
Ahmed, who has long been interested in modifying biology through chemistry, said it’s important to be able to miniaturize processes to make them smaller and more convenient. It's the same concept as a computer, he said.
“When computers were first invented,” he said, “it filled a whole room. But now you have a phone that fits in your pocket that’s even more powerful. We want to be able to do this tagging with the smallest possible probe. It opens doors to new things that you can do.”
The key, he said, is that this is the closest anyone has gotten to an amino acid. Although people have made derivatives of amino acids before, they’re much larger, whereas this one is just two atoms larger.
Using this fluorescent reporter, scientists may be able to do experiments observing just one molecule at a time to reveal dynamics that would otherwise be hidden.
Single molecule experiments have never been done with amino acid-based fluorophores, which will allow researchers to label proteins with a smaller fluorophore than those currently used in the field.
According to Hilaire, this new fluorophore will allow scientists to learn more and explore many different avenues.
“If you talk to anyone in biology,” Gai said, “the discovery and application of GFP really revolutionized the field just because suddenly we could see new things. But one thing cannot do everything, so we still really need new probes that have different sizes, colors and properties which will allow us to apply to study a wide variety of biological processes which are all different and require different methods. We think that many people will pick up what we have done and either extend the design to make better fluorophores, or just utilize it to do their own research.”
This work was made possible through funding from the National Institutes of Health and can be found here.
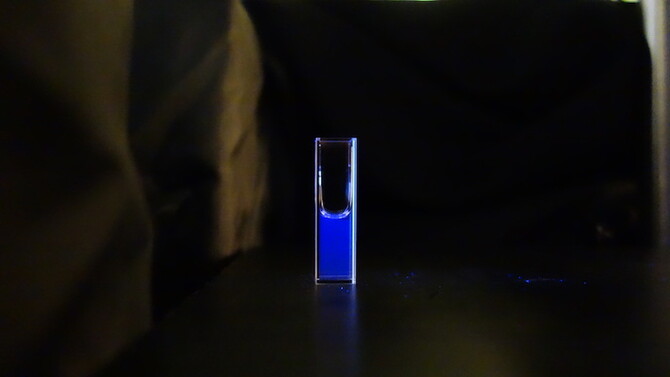