Penn Researchers Uncover a New Way Heat Travels Between Molecules
A new model, developed by University of Pennsylvania chemists, could be the first step towards better harnessing heat energy to power nanoscale devices.
Scientists have long understood that heat travels through vibrations. Molecules vibrate faster and faster as they heat up, and their vibrations cause other molecules around them to vibrate as well, warming cooler nearby molecules. For decades this was the only known way heat could be transferred in organic molecules. Only recently have researchers had the ability to take a closer look at what actually happens at the molecular scale during heat transfer.
Abraham Nitzan, professor of chemistry in Penn’s School of Arts & Science, and Galen Craven, a postdoc in his lab, used new information about how to measure temperature on a nanoscale to revisit the mechanism of heat transfer. They created a model to find out how a temperature gradient affects molecular interaction, focusing in on the process of electron transfer.
Their findings, published in the Proceedings of the National Academy of the Sciences, show that heat transfer occurs when the electron moves between two molecules that are at different temperatures.
Electron transfer is possibly the most important process in chemistry, according to Nitzan.
“Half of chemistry is electron transfer processes,” he said. “It has been investigated for 100 years on the molecular scale.”
Electrons, the negatively charged component of atoms, orbit a positively charged nucleus. In metals, electrons can move freely from molecule to molecule, producing an electric current. Electron transfer in organic molecules, however, requires more energy. When a molecule is energized, an electron will “jump” from one molecule to orbit another. This electron transfer process is essential for many common chemical reactions, especially ones that occur in biological processes.
While electron transfer has been meticulously studied, only recently have scientists been able to look at temperature on the scale of atoms and electrons. Today, scientists can detect temperature differences on the scale of a few nanometers, allowing them to see how differences across individual molecules affect their behavior.
This innovation is what inspired Nitzan and Craven to investigate how heat transfer occurs at the molecular level.
“The question we wanted to answer,” said Craven, “is what happens when the donor and the acceptor are at different temperatures.”
Nitzan and Craven made a series of mathematical equations to describe exactly that. Building on the experimental results obtained using new tools to measure heat differences over very small distances, they created a theory of how electrons jump to molecules with less heat energy. Their model shows that heat transfer does in fact occur when an electron transfers to a lower temperature molecule. They also observed that, compared to heat transfer via vibration, electron transfer could move heat as much as a million times faster.
Craven believes this could be a key discovery to improve the efficiency of nanotechnology devices that rely on small-scale interactions to operate. On the nanoscale, the movement of energy from a molecule with more heat to one from less could be harnessed to power emergent technologies and devices.
For instance, Craven imagines that computers could be designed to use heat rather than electricity to perform logical operations. In the past, such computers would be impossible because vibrational heat transfer is too slow and would not generate enough power to run.
But, “if we’re using the electron’s speed to move heat,” said Craven, “we could have these computers operating at the speeds of electrical computers but using heat instead of electrical currents.”
Unlike battery power, which uses a difference in electrical charge to generate energy, a computer that uses heat gradients for power could have advantages. For instance, it could be used in extreme environments without fear of short-circuiting.
The Penn researchers remain cautious, however, about the promise of applying this knowledge until their theory is further developed, noting that, for an electron to carry heat, it must be strongly associated with the vibration of the molecule so it can carry some of that vibrational energy when it jumps to another orbit. While electron transfer alone can travel as much as a million times faster, they noted that these vibrations may cause the electrons to transfer more slowly.
“The more strongly electrons coupled to the vibrations, the smaller the electron speed will be,” said Nitzan. “Electrons with strong coupling to vibrations carry a lot of heat, but strong coupling also slows you down. There will be a balance between the two, and this is something to investigate in the future.”
This model, however, is a new discovery of a fundamental process that will change our understanding of how heat transfer works on a molecular level.
“Eventually what we envision in nanotechnology is energy flow and charge transfer on the nanoscale,” said Nitzan, “so it is very important to properly know and understand how molecules interact.”
Both Nitzan and Craven hope to work on using this theory for applications in nanotechnology in their future research.
The Israel Science Foundation, the US–Israel Binational Science Foundation and the University of Pennsylvania provided funding for this project.
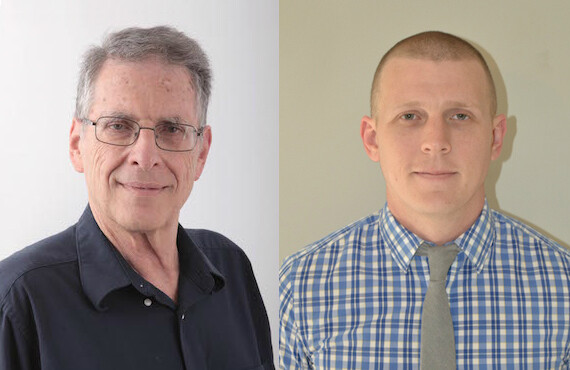