Penn Researchers Use ‘Soft’ Nanoparticles to Model Behavior at Interfaces
Where water and oil meet, a two-dimensional world exists. This interface presents a potentially useful set of properties for chemists and engineers, but getting anything more complex than a soap molecule to stay there and behave predictably remains a challenge.
A University of Pennsylvania team has now shown how to make nanoparticles that are attracted to this interface but not to each other, creating a system that acts as a two-dimensional liquid. By measuring this liquid’s pressure and density, they have shown a way forward in using it for a variety of applications, such as in nanomanufacturing, catalysis and photonic devices.
By creating a system where these particles do not clump into clusters or skins, they have enabled a way of investigating the physical fundamentals of how nanoscale objects interact with one another in two dimensions.
The work was conducted by postdoctoral researcher Valeria Garbin, graduate student Ian Jenkins and professors Talid Sinno, John Crocker and Kathleen Stebe, all of the Department of Chemical and Biomolecular Engineering in Penn’s School of Engineering and Applied Science.
It was published in Physical Review Letters.
“Things get stuck at the interface between oil and water,” Stebe said. “That’s of tremendous fundamental and technological interest, because we can think of that interface as a two-dimensional world. If we can start to understand the interactions of the things that accumulate there and learn how they are arranged, we can exploit them in a number of different applications.”
Getting nanoparticles to go to and stay at this interface is tricky, however. Their surface chemistry can easily be adapted to either water or oil, but balancing the two to get the particles to stay in this 2-D regime is more difficult.
“We understand how particles work in 3-D,” Crocker said. “If you put polymer chains on the surface that are attracted to the solvent, the particles will bounce off each other and make a nice suspension, meaning you can do work with them. However, people haven't really done that in 2-D before.”
Even when particles are able to stay at the interface, they tend to clump together and form a skin that can’t be pulled apart into its constituent particles.
“All particles love themselves,” Stebe said. “Just due to Van der Waals interactions, if they can get close enough, they aggregate. But because our nanoparticles have protective ligand arms, they don’t clump together and form a liquid state. They’re in two-dimensional equilibrium.”
The team’s technique for surmounting this problem hinged on decorating their gold nanoparticles with surfactant, or soap-like, ligands. These ligands have a water-loving head and an oil-loving tail, and the way they are attached to the central particle allows them to contort themselves so both sides are happy when the particle is at an interface. This arrangement produces a “flying saucer” shape, with the ligands stretching out more at the interface than above or below. These ligand bumpers keeps the particles from clumping together.
“This is a very beautiful system,” Stebe said. “The ability to tune their packing means that we can now take everything we know about the equilibrium thermodynamics in two dimensions and start to pose questions about particle layers. Do these particles behave like we think they should? How can we manipulate them in the future?”
To get at the fundamentals of this system, the researchers needed to deduce the relationships of certain properties, such as how the pressure of their 2-D liquid changes as a function of the packing of the particles. They used a variation of the pendant drop method, in which an oil droplet formed in a suspension of particles in water. Over time, particles attached to the oil-water interface, producing the 2-D liquid in a form where they could measure those traits.
“We can infer the pressure of this 2-D fluid by the shape of the drop,” Stebe said. “Once we compress the drop by pulling some of the oil back into the syringe, we can determine how the shape changes and relate it to the pressure in the layer.”
The researchers also needed to determine how densely the particles were packed. To do so, they wanted to take advantage of the fact that the drop became more opaque as the density of the particle increased when the drop was compressed. However, it was not possible to simply measure the amount of light that shone through the drop, as plasmonic behavior meant that the properties of the gold nanoparticles changed as they got closer together.
“Fortunately, we discovered another interesting feature of this nanoparticle system,” Garbin said. “If the drop was compressed too much, some particles would fall out of the interface because they didn't fit anymore. This enabled us to measure the amount of particles that were in that falling plume, since the particles are farther apart from each other there. From that measurement, we could work backwards to the number of particles on the interface”
The smooth relationship between the particles’ packing and the pressure of the 2-D liquid they form provides the basis of universal rules that govern the physics of such systems.
”From this data,” Crocker said, “we can figure out the force versus distance of two nanoparticles. That means we can now make a model of how these particles behave in the 2-D liquid.”
Having these rules will allow researchers to develop functional nanoparticles with different traits, such as longer and more complex ligands that perform some chemical task.
“One application is interface catalysis,” Stebe said. “For example, if you have a reagent that’s in the oil phase, but its product is in the water phase, having a particle on the interface that can help move it from one to the other would be perfect.”
A better understanding of when and why particles get trapped in liquid-liquid interfaces could also underpin future work.
Valeria Garbin is now an assistant professor in the Department of Chemical Engineering at Imperial College London.
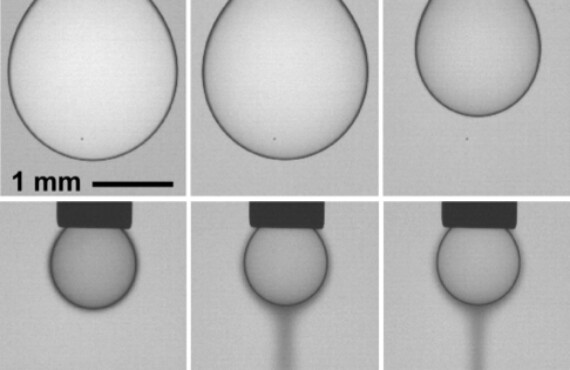