Penn collaboration works to answer a fundamental nanotechnology question
Physicists at the University of Pennsylvania have invented a new type of graphene-based sensor that could one day be used as a low-cost diagnostic system able to test for biomarker molecules, which are indicative of disease states.
In collaboration with Penn chemists, the researchers published a paper in Chemical Science in which they use this sensor, for the first time, to directly answer a basic scientific question in nanotechnology about whether a particular protein maintains its structure when it assembles around an inorganic nanoparticle. The finding may have medical applications down the line.
The research was led by Jinglei Ping, a postdoctoral researcher in the Department of Physics & Astronomy in Penn’s School of Arts & Sciences, physics professor A.T. Charlie Johnson and Katherine Pulsipher, previously a grad student in the Department of Chemistry and now a postdoc in the Department of Chemical and Biomolecular Engineering in the School of Engineering and Applied Science. Chemistry professors Ivan Dmochowski and Jeffery Saven, graduate students Ramya Vishnubhotla and Jose Villegas and alumnae Tacey Hicks and Stephanie Honig also contributed to the study.
This unique sensor, which the researchers call a graphene micro electrode, works by measuring the current that flows from a liquid sample to the graphene surface. The researchers found that this technique is especially useful in more complicated liquid samples, such as biological fluids, which typically have a lot of salt and proteins.
Dmochowski’s group has been studying the interaction of inorganic nanoparticles with proteins for the past decade. Specifically, the researchers have been looking at a type of protein called ferritin, which is better known for being an iron-storage protein. A particular thermophilic ferritin has the unusual feature of assembling in high salt and disassembling in low salt.
“What we found some years ago,” Dmochowski said, “is that we could take salt out of the equation and work in low salt where the protein would normally be disassembled and put in a gold nanoparticle with a coating that makes it very charged in solution with the ferritin. The nanoparticle is spontaneously encapsulated by the protein, like a ship in a bottle. The protein cloaks the nanoparticle and changes its properties in solution.”
Inorganic nanoparticles are widely considered potential next-generation diagnostic and therapeutic agents. However, they are known to interact with and denature proteins, which form a thin shell around the nanoparticle called a corona.
“That's not what happens with our protein,” Dmochowski said. “It has 24 different subunits that come together to encapsulate the nanoparticle, and we have found over the years that it maintains much of its native structure.”
But the researchers had an unresolved question. One characteristic feature of this protein is that is has four triangular nano-sized pores. The researchers wanted to know whether these pores are maintained when the protein assembles around the gold nanoparticle. Although there are several techniques that might be able to resolve this challenging imaging question, Johnson said, they tend to be a bit more indirect and cumbersome.
Through discussions with Dmochowski, Ping and Johnson realized they could use this new graphene sensor to try to answer this question.
“We were trying to think about something different that we could do with our sensor,” Johnson said, “and we came up with the idea that we could use it to learn something about this bio-nanosystem. We basically brought together my group's work on graphene circuits and devices with Ivan’s group’s work on creating ferritin-nanoparticle assemblies and new mutant types of ferritin.”
The researchers made a different type of molecule that they knew had no pores and compared it to the “wild type” that exists in nature and does have pores. They measured the current flowing from the solution into this micro electrode. They saw that in the wild type there was current flowing via the metal particle. When there were no pores, the current was “blocked off” and could not flow via the metal particle. By using this new technique, the researchers were able to show that the pores were maintained in the wild type.
The protein-encapsulated gold nanoparticles that were used in this study are showing promise as useful building blocks for chemical, physical and medicinal purposes, Dmochowski said.
The protein is interesting for nanotechnology as well. In the body, ferritin surrounds a small iron particle and transports it around, but it can be manipulated to encapsulate other particles in a way that makes them biocompatible.
“This research could provide fundamental building blocks that you would then integrate with many of these types of protein-nanoparticle assemblies to make devices,” said Dmochowski. “The fact that these pores are maintained was important to confirm because it gives some control over what species can interact directly with the gold nanoparticle.”
It should be possible, he said, to take those same ferritin shells and, instead of a nanoparticle, put a therapeutic protein inside and crosslink the ferritin.
“In that scenario, it’s protecting another protein,” he said, “and then you can functionalize the outside of the ferritin to direct it to places it wouldn't normally go in the body. There's obvious potential for delivering therapeutic nanoparticles, proteins or other cargo using these types of ferritin scaffolds.”
Johnson’s group is moving ahead to try to look for applications of this new sensor in the world of low-cost advanced diagnostics. A real challenge to doing high-quality measurements in real world samples is that these samples tend to be more complicated. This paper both validates that their method works in these complicated samples and shows some utility for their technique.
“The sensor works well in solutions that are salty,” Johnson said, “which is an advantage, and we have another paper that's been published where we showed that it can work in solutions such as human serum which is, relatively speaking, very complicated. We're headed towards looking at this as a new, hopefully superior type of nanosensor for use in real biological samples such as blood.”
Dmochowski highlights that this research is a great example of the type of science that can be conducted on Penn’s compact campus where there exists high-quality basic science research, engineering research and medical research, all happening within one or two blocks.
“A lot of it comes down to having the clearest view yet of what these proteins look like when they assemble around the nanoparticle,” Dmochowski said. “We've been studying this for a long time, and it's often the case in chemistry you have to come up with new methods of studying new types of matter. In this case, Charlie's group had the perfect technique for looking at this. We learned a lot through interacting with people in physics, and I think they learned a lot from us. It was very synergistic, and it helped us to shed more insight about how these proteins interact with each other at the gold surface.”
This research was supported by the Defense Advanced Research Projects Agency and the U.S. Army Research Office under grant number W911NF1010093 and National Science Foundation grant CHE-1508318.
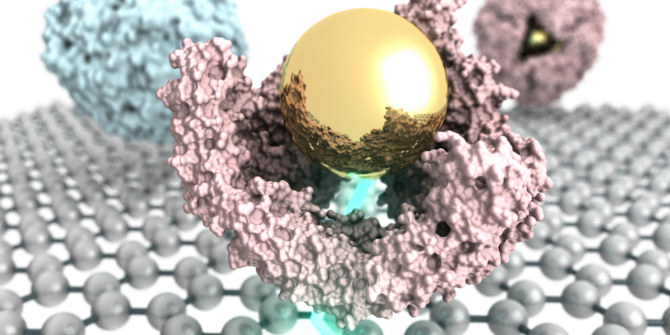