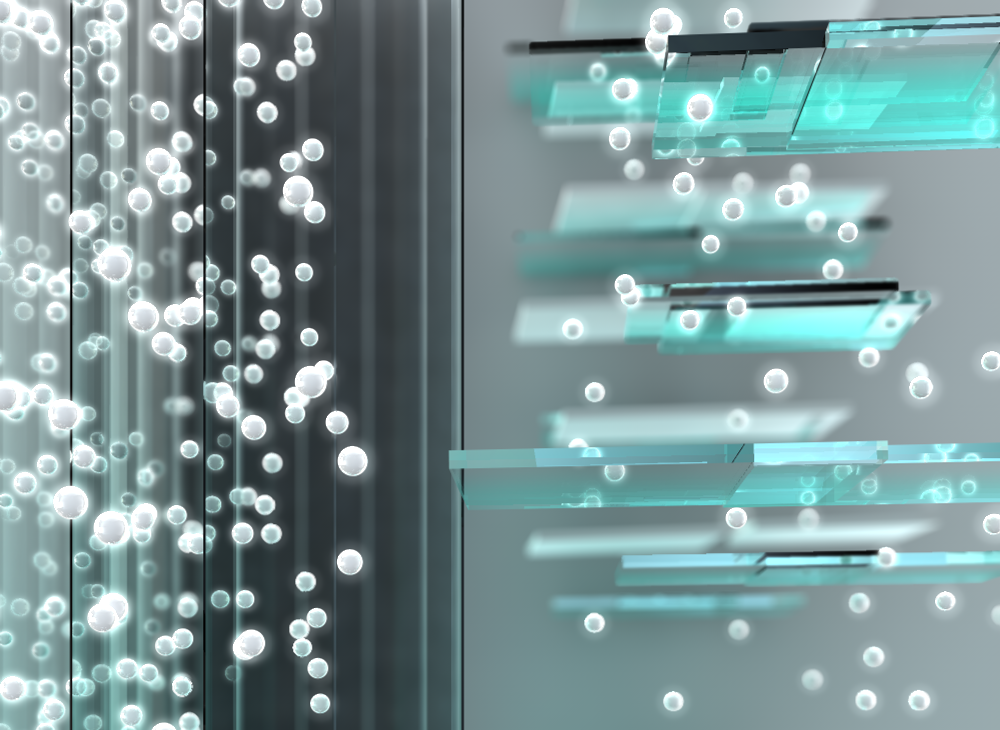
When it comes to cell phones, there are few things more frustrating than a short battery life. As the battery bar of a phone dwindles down below 10 percent, there’s a mad rush to find a charger and an outlet, and then it can take up to an hour for the battery to fully charge. Twelve hours later, the process repeats when the battery drains itself once again.
But researchers at the University of Pennsylvania and Drexel University are working together on a novel technique that will allow batteries and supercapacitors to store more energy and last longer as well as drastically reduce the amount of time they take to fully charge. The technique could lead to better phones and electric cars, and even wearable chargers woven into the fabric of a shirt. Their most recent results, which focused on supercapacitors, have been published in Nature.
According to lead author Yu Xia, there’s been a huge demand from industry for a way to develop new battery and capacitor systems with higher energy density (how much energy the devices can store) and power density (how fast the device can charge.) Researchers have been working on using two-dimensional nanomaterials that are atomically thin but a few hundred nanometers to microns in width, to accomplish this.
The problem is that these nanosheets tend to stack up horizontally like sheets of paper in a book. This results in a prolonged ion diffusion length that causes a huge suppression of their performances when the thickness of materials in the electrode approaches industrial standards. So when they are stacked up to 100 micrometers thick, the industry standard for energy devices, the materials lose their functionality.
“The ion diffusion problem in energy storage devices,” Xia says, “has been long recognized as one of the major obstacles impeding the industrial development of new batteries and supercapacitors with higher energy and power density.”
But the Penn and Drexel researchers have a completely new idea about how to tackle this problem. Xia, a postdoctoral fellow in Penn Professor Shu Yang’s lab approached a Drexel team in Professor Yury Gogotsi’s lab about aligning 2-D nanomaterials using a process called soft matter self-assembly. The researchers work with MXenes, a new class of nanomaterials discovered by Drexel University researchers and developed in Gogotsi’s lab, which are particularly well-suited for energy storage. Unlike graphene, which is only a 2-D layer of carbon, MXenes are much richer in chemical compositions, and thus, functions.
“About 30 MXenes have already been made, millions of compositions are possible, and at least a few MXenes can be made in 100-gram batches in our lab,” says Yury Gogotsi. “This is what makes them promising for large-scale industrial applications.”
Here, the researchers found a way to assemble the 2D titanium carbide MXene into liquid crystalline phases.
Soft matter self-assembly is a process that has been around for billions of years. It's one of the key modes through which nature constructs living matter. The assembly process used by the Penn and Drexel teams, however, is unique: they make the atomically thin sheets stand up vertically using a familiar process—a process that drives the liquid crystal displays on phones, televisions, and laptops. This process, called alignment in the liquid crystalline phase, involves a special phase between the crystalline solid and the disordered liquid.
“The major novelty of our approach,” Xia says, “is that we are able to align the MXene flakes in the vertical direction, so the ion diffusion in the supercapacitors could be extensively accelerated, leading to the thickness-independent supercapacitors. Our design relied on the unique assembly of MXene into a long-range ordered, liquid crystalline phase using soft forces, allowing us to align them vertically nearly effortlessly. Directing the alignment of functional nanomaterials has just begun. Our work reported here demonstrates how effective it is in energy storage.”
Although a couple of research groups have attempted to engineer vertical alignment using a top-down process, it’s difficult to scale up for industrial applications.
“Our process is through self-assembly,” Yang says, “so it’s much cheaper and can be scalable over a large area. In the end, it’s the concept of how to bring our knowledge in soft forces that are abundant in nature to align highly engineered, hard materials with interesting nanostructures and functionality.”
To get the 2-D material to undergo this process, the researchers used a surfactant, which can squeeze between the 2-D nanosheets and help them form a liquid crystal phase. The researchers then applied a mechanical shearing method to it, which forced the molecules to orient in the vertical direction, indeed a preferred orientation, within which ions could have directional diffusion that has been found only weakly depends on electrode thickness. Since the ions can travel directly from top to bottom, the researchers are able to make the 200-micron thick electrodes while still maintaining their functionality.
“Liquid crystals are the original nanomaterials,” Yang says. “When speaking of liquid crystals, people often think of liquid crystal displays, a more than half-century old technology. But liquid crystal is a phase. Anything that has an isotropic shape, such as the 2-D nanomaterials, can be assembled into a liquid crystal phase. Attracted to the beauty and richness of the liquid crystal phases, we have been working on this for the last eight years, within the interdisciplinary research group at the Laboratory for Research on the Structure of Matter, the National Science Foundation supported Materials Research Science & Engineering Center at Penn. We just keep discovering new ways to manipulate them to create new functional materials.”
This paper focused on the use of this method in supercapacitors, but the researchers hope to follow up by applying the same principles to batteries, catalysts, and solar cells. They are also trying to extend this technique to other MXenes, as well as other 2-D nanomaterials, such as graphene. Although the researchers acknowledge that there are other challenges to overcome before the method can be used in real world devices, they believe their findings provide an exciting leap forward in the field. The long-term goals are to apply the method to mobile electronic devices, electric cars, and renewable energy harvesting technologies.
“It's a perfect marriage between soft matter self-assembly forces and functional hard nanomaterials,” Xia says. “After more than a decade of active research in 2-D materials, we present a possible way to overcome one of the biggest barriers to application in energy storage devices and are actually creating a system that is one of the most plausible ways to push these nanomaterials into industry. This is very exciting.”
Yu Xia is a postdoctoral fellow in the School of Engineering and Applied Science at Penn.
Shu Yang is a professor the in the Departments of Materials Science and Engineering and Chemical and Biomolecular Engineering in the School of Engineering and Applied Science at Penn.
Yury Gogotsi is the Director of the A.J. Drexel Nanomaterials Institute and Distinguished University and Charles T. and Ruth M. Bach Professor in the Department of Materials Science and Engineering at Drexel University.
This research was supported by the National Science Foundation and the U.S. Department of Energy.