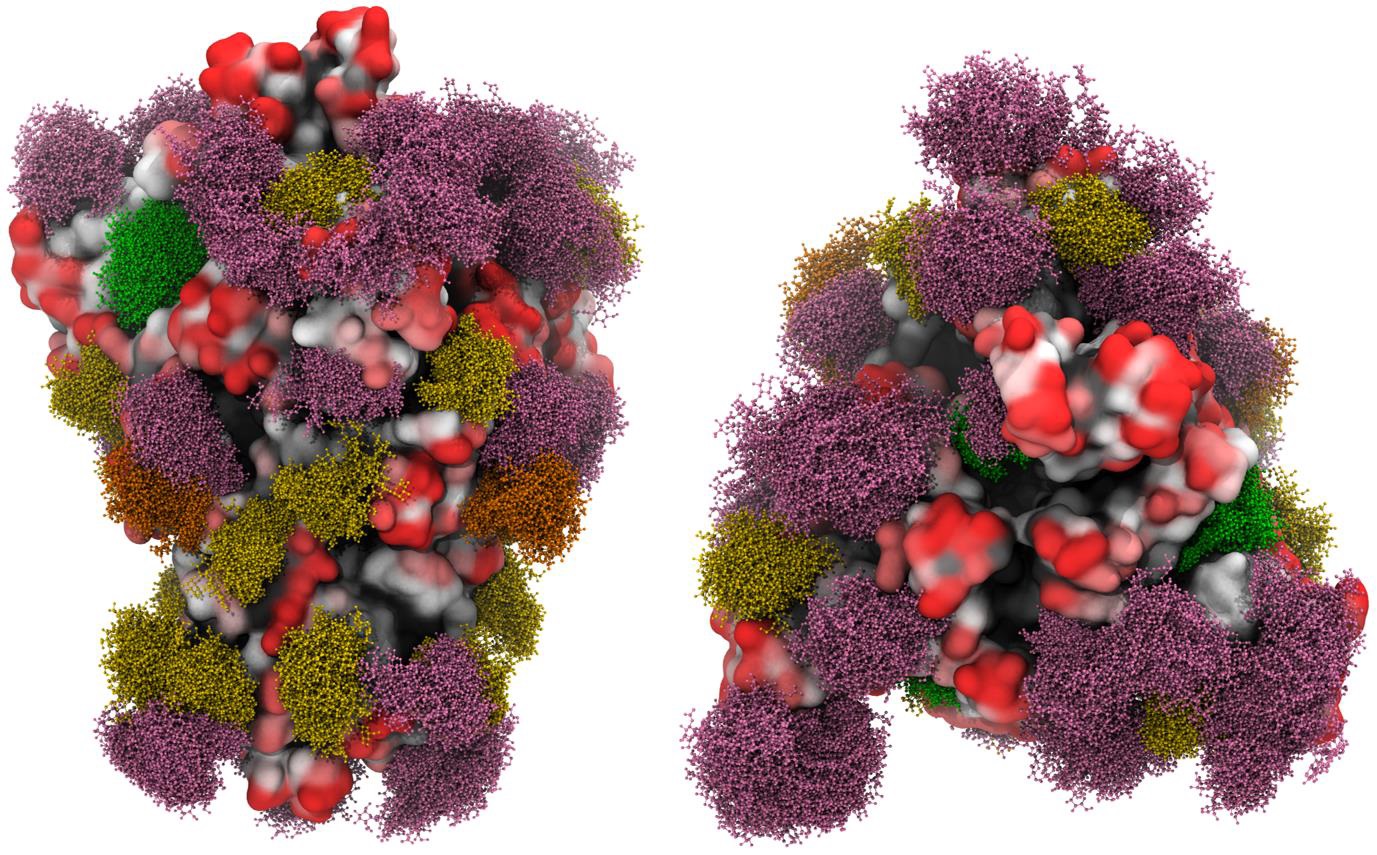
Research published in the Proceedings of the National Academy of Sciences describes a new approach for creating synthetic cell membrane mimics. Using a new approach for “click” chemistry, researchers designed self-organizing nanovesicles that can have their surfaces decorated with similar sugar molecules as viruses, bacteria, or living cells. The result of a collaboration between Penn, Temple University, the Max Planck Institute, the Leibniz Institute for Interactive Materials, RWTH Aachen University, and Freie Universität Berlin, this work provides a new tool for studying how certain pathogens, such as the novel coronavirus, can evade detection by a host’s immune system.
The outer layers of cells are decorated with proteins, lipids, and sugar molecules that are used for communicating with and recognizing other cells. One of the most common types of sugars is mannose, which is found in long, branched chains and is also connected to other biomolecules. These oligomannoses are also commonly found on bacteria and viruses and are thought to help pathogens avoid being detected by a host’s immune system; however, the precise way that these sugars help pathogens evade detection is not well understood.
One approach to study cellular interactions is to create synthetic cells that can have customized sequences of sugars or proteins, allowing scientists to answer specific questions about how cells work. Previous research by the same team used these synthetic cells to better understand how short-chain sugars act as “communication channels.” Now, using these same cell mimics, the researchers were interested in understanding the role of complex sugars, including oligomannose, which are more akin to what’s prevalent on cell and pathogen surfaces.
But in order to do this the researchers needed a new way to create cell mimics that could contain the types of complex sugar molecules they were interested in studying. The methods currently used to create these synthetic cells made it difficult to attach a water-loving, hydrophilic sugar molecule since the intermediate required by the method has to be hydrophobic. In addition, the methods used in the lab of Peter Seeberger are only able to synthesize a few milligrams of synthetic sugars at a time.
To address both these challenges, the researchers developed a new approach to synthesize their cell mimics through “click” chemistry, where two smaller molecules are linked together to form a larger molecule that only requires a small amount of starting material. They found that an isothiocyanate-amine reaction, used for protein sequencing but never before for click chemistry, was able to add large sugar molecules to the outsides of the synthetic cells. “That provided us with a tool to investigate these sugars on the surface of a cell-like assembly,” says Virgil Percec. “You make the hydrophobic character to become hydrophilic and provide the structure that we could not have accessed through other chemistry.”
With their new tool in hand, the researchers used methods previously developed by the team to study their self-assembled nanostructures, including cryo-EM to visualize the cell mimics and AFM to study their surface morphology. By looking at a subset of cell mimics that contained oligomannose sequences similar to that of a typical cell, they found that longer sugars could bind to other cell surfaces with more strength and greater efficiency.
Viruses are covered in oligomannoses. One example is the binding protein on coronavirus, known as the spike protein, which is decorated with so-called “camouflage” oligomannoses, which are thought to help the virus avoid detection and inactivation by a host’s immune cells. Thanks to their new study, the researchers now hope to learn more about how viruses like SARS-CoV-2 are able to use these sugar molecules to hide from immune cells. “These synthetic nano-scale mimics are a tool to investigate the behaviors of these sugars and how they might interact,” adds Michael Klein about the implications of their recent finding.
If their vesicles are shown to be safe in animal models, the researchers could also explore ways to use these cell mimics to deliver therapeutics. While that research goal would be something to aim for in the near future, the team remains eager to get back into the lab and continue their fundamental yet impactful research. “This is a very fundamental question that we were asking at the beginning that is now of general interest to the problem that we have today,” says Percec. “We are working with a tool that hopefully will go very fast from now on.”
Virgl Percec is the P. Roy Vagelos Professor of Chemistry in the Department of Chemistry in the School of Arts & Sciences at the University of Pennsylvania.
This research was supported by National Science Foundation grants DMR-1807127 and DMR-1720530, the P. Roy Vagelos Chair at Penn, and the Alexander von Humboldt Foundation.