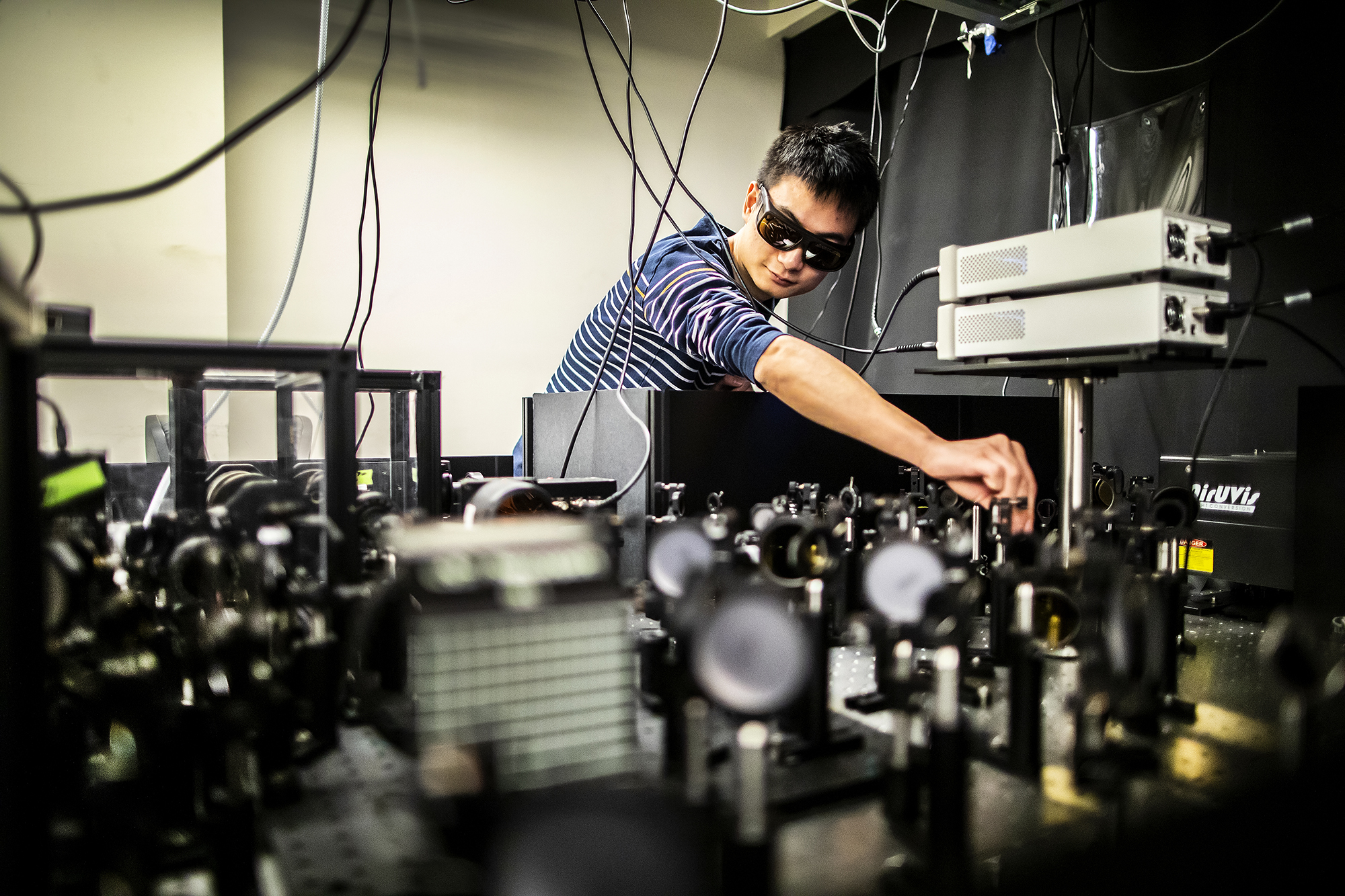
A new study provides the first evidence of exotic particles, known as fourfold topological quasiparticles, in the metallic alloy cobalt monosilicide. Published in the Proceedings of the National Academy of Sciences, this comprehensive analysis, one that combines experimental data with theoretical models, provides a detailed understanding of this material. These insights could be used to engineer this and other similar materials with unique and controllable properties. The discovery was the result of a collaboration between researchers at Penn, University of Fribourg, French National Centre for Scientific Research (CNRS), Max Planck Institute for Chemical Physics of Solids, and University of Maryland.
The theories underpinning topological insulators, materials with a conductive surface and an insulating core, were pioneered by Penn’s Charlie Kane and Eugene Mele, winners of the 2019 Breakthrough Prize in Fundamental Physics. Through their theoretical contributions on topology and symmetry, Kane and Mele postulated the existence of this new class of materials, ones that could be used to create high-efficiency electronics or quantum computing platforms.
“But the desire of all theorists is for their work to translate into the real world,” says chemist Andrew M. Rappe, who collaborates with Kane and Mele on ways to discover real-world materials that have these exotic properties. “The recent hiring of Professor Liang Wu takes our topological physics group to a new level, one where we can understand the materials and observe their properties, all in a close, collaborative loop.”
Since coming to Penn in 2018, Wu and his lab have used optics experiments to study how light interacts with topological materials and are interested in validating some of the existing theories about this class of materials. Last year, graduate student Zhuoliang Ni was conducting light pulse laser experiments with cobalt monosilicide (CoSi) to better understand the relationship between topology and nonlinear optics and to see if they could use this material to convert light into electric current. The data they collected seemed to suggest that there might be some unique topological features of CoSi. “I realized that there’s something interesting in the optical conductivity by itself,” says Wu, who then reached out to Mele and Rappe about developing a theory to help explain the results of their experiment.
While CoSi had been studied before, the new data collected by Wu’s lab was of a higher quality than previous work, allowing the researchers to develop a model that provided a more robust explanation of their findings.
“The predictions of topological physics suggested that this material should have some exciting properties, such as linear optical conductivity with increasing photon energy, but a real material has many phenomena going on at the same time,” says Rappe. “Theorists gradually make their model more complicated and realistic, and the experimentalists account for other features to simplify the experimental presentation. That’s how we come to agreement about which features can be ascribed to the topological properties.”
After nearly a year of analyzing data and iterating on different theories, one of the things that stood out was how well these models, ranging from simple to complex, agreed with one another. “It’s surprising to see this level of agreement for ourselves,” says graduate student Zhenyao Fang, who led the theoretical part of this study. “Some models are purely derived from physical theories, and some are numerical models derived from first principles methods, so it’s surprising to observe this kind of agreement between them.”
Now, thanks to a combination of cleaner data and robust theoretical models, this cohesion between the theory and the experiments demonstrated in this paper represents a huge step forward, says Wu. “The agreement between experiment and theory is extremely good,” he adds. “Here we provide an example of a comprehensive combination of experiment and theoretical understanding, and this can be applied to many other new materials or systems that will be discovered in the future.”
Because CoSi is in a family of materials with a very common crystal structure, the material could be used in alloys with magnetism that are engineered to have more complex topological magnetic properties because of an ability to control their design atom by atom.
This work is also a showcase of Penn’s expertise in topological physics and paves the way for future experimental and theoretical progress in this field at the University, says Rappe. “We now have a vibrant group that merges efforts in topological electronics and photonics,” he says. “Topological physics is growing, and we’ve blazed a trail that other people can follow with other materials for designing desirable opto-electronic properties.”
Andrew M. Rappe is the Blanchard Professor in the Department of Chemistry in the School of Arts & Sciences at the University of Pennsylvania.
Liang Wu is an assistant professor in the Department of Physics and Astronomy in the School of Arts & Sciences at the University of Pennsylvania.
This research was supported by the Army Research Office (Grant W911NF1910342), National Science Foundation (Grant DMR-1720530), and U.S. Department of Energy Office of Basic Energy Sciences (Grant DE-FG02-07ER46431).
The complete list of co-authors includes Bing Xu, Zhenyao Fang, Miguel-Ángel Sánchez-Martínez, Jorn W. F. Venderbos, Zhuoliang Ni, Tian Qiu, Kaustuv Manna, Kefeng Wang, Johnpierre Paglione, Christian Bernhard, Claudia Felser, Eugene J. Mele, Adolfo G. Grushin, Andrew M. Rappe, and Liang Wu.