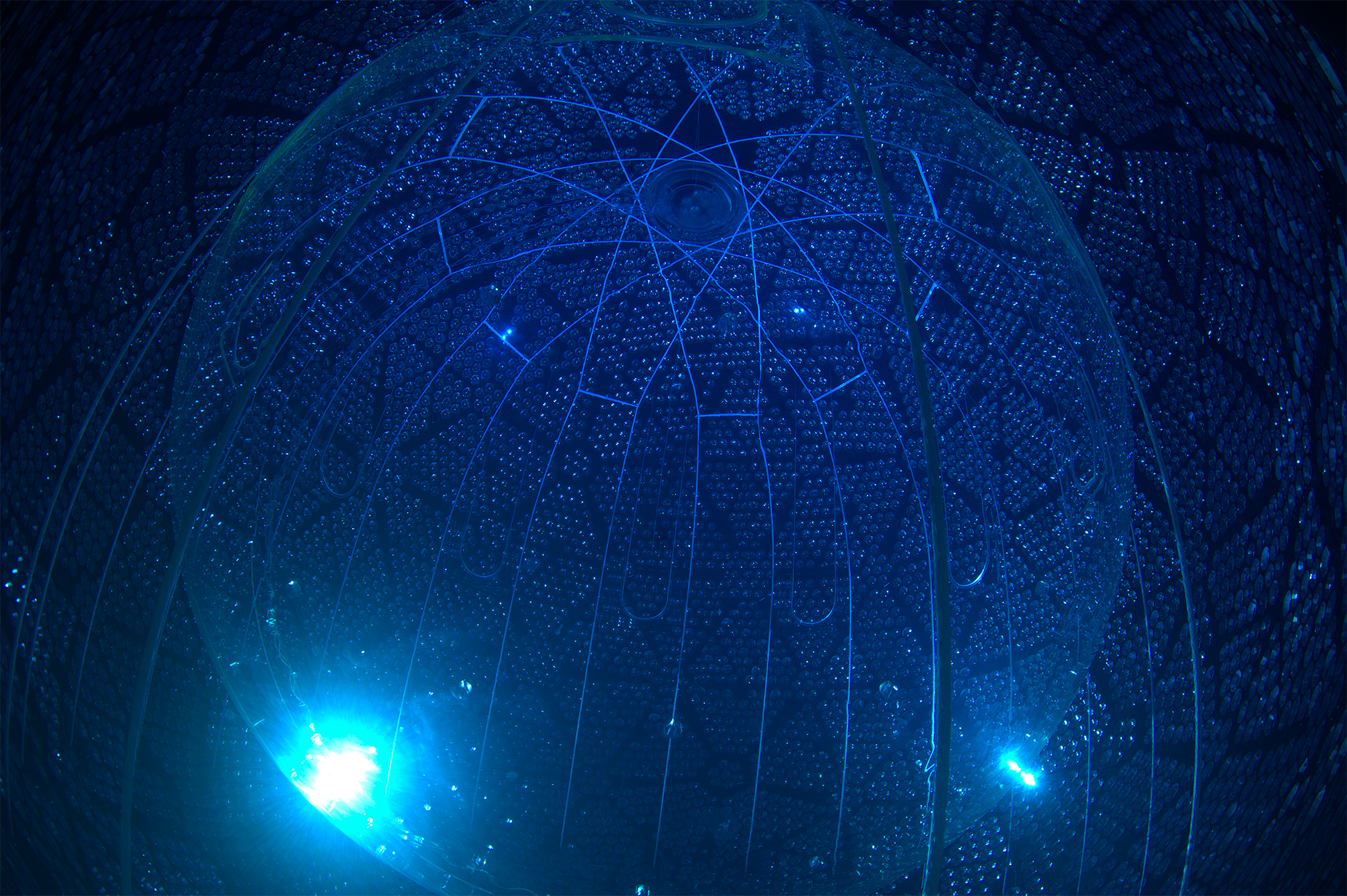
Neutrinos are extremely lightweight subatomic particles that are produced during nuclear reactions both here on Earth and in the center of stars. But neutrinos aren’t harmful or radioactive: In fact, nearly 100 trillion neutrinos bombard Earth every second and usually pass through the world without notice.
Joshua Klein is an experimental particle physicist who studies neutrinos and dark matter. His group, along with retired professor Eugene Beier, collaborates with the Sudbury Neutrino Observatory (SNO), an international research endeavor focused on the study of neutrinos. Klein and Beier’s groups previously designed and now maintain the electronics at SNOLAB that collect data on these subatomic particles.
Klein is fascinated by neutrinos and how they could help answer fundamental questions about the nature of the universe. “They may explain why the universe is made up of matter and not equal parts matter and anti-matter, they may be responsible for how stars explode, they may even tell us something about the laws of physics at the highest energy scales,” says Klein.
Previous research on neutrinos has already led to groundbreaking discoveries in particle physics. The SNO collaboration was awarded the 2016 Breakthrough Prize in Fundamental Physics for solving the “solar neutrino problem.” The problem was that the number of neutrinos being produced by the sun was only a third of what was predicted by theoretical physicists, a discrepancy that had puzzled researchers since the 1970s.
To solve this, researchers went about 1.2 miles underground to study neutrinos in order to avoid the cosmic radioactive particles that could interfere with their minute and precise measurements. The SNOLAB facility in Sudbury, Canada, which houses a a 40-foot wide acrylic vessel surrounded by photodetectors, allowed physicists to measure the three different types of neutrinos at the same time. Physicists found that neutrinos were able to change from one type into another.
Today, 15 years later, researchers are looking for an incredibly rare process involving neutrinos that, if found, could revolutionize the field of fundamental physics. “Now that we know that neutrinos can change form, along with the fact that neutrinos have mass but no charge, we can hypothesize that they can be their own antiparticle. If this is true, it could explain why the universe is made of only matter,” says Klein.
The question of what happened to all the antimatter has remained unanswered since Paul Dirac won the Nobel Prize in 1933 after calculating that every particle in the universe must have a corresponding antiparticle. But the majority of the universe is made of ordinary matter, not equal parts matter and anti-matter, and scientists are trying to figure out why.
The photodetectors at SNOLAB are now being upgraded as part of SNO+ in order to search for a rare type of radioactive decay known as a neutrinoless double beta decay, a never-before seen process that would prove that neutrinos and anti-neutrinos are actually the same particle. Witnessing a neutrinoless double-beta decay event is so rare, if it even exists, and would give off such a small signal that the only way to detect it is through the combination of powerful equipment, refined analyses, and a lot of patience.
Instead of sitting around waiting for a rare event to happen, researchers are actively taking advantage of this state-of-the-art underground facility. “One of the selling points of SNO+ is that it’s a multipurpose detector,” says graduate student Eric Marzec. “A lot of detectors are produced with a singular goal, like detecting dark matter, but SNO+ has a lot of other interesting physics that it can probe.”
Here at Penn, students from the Klein lab conduct key maintenance and repairs on the electronic components that are instrumental to the success of SNO+. They also conduct research on new materials that can help increase the sensitivity of the detector, providing more chances of seeing a rare neutrinoless double-beta decay event.
Marzec and Klein were part of a recent study using SNO+’s upgraded capabilities to collect new data on solar neutrinos. Before the detector vessel is filled with scintillator, a soap-like liquid that will help them detect rare radioactive decays, it was briefly filled with water. This enabled researchers to collect data on what direction the neutrinos came from, which then allowed them to focus their efforts on studying neutrinos that came from the Sun.
The solar neutrino problem may be solved, but new data on solar neutrinos is still incredibly useful, especially since data from SNO+ have very low background signals from things like cosmic radiation. “There’s only a few experiments that have ever been able to measure neutrinos coming from the sun,” says Marzec. “People might someday want to look at whether the neutrino production of the sun varies over time, so it’s useful to have as many time points and as many measurements over the years as possible."
Marzec has spent a considerable amount of time working at the SNOLAB facility in northern Ontario. He describes a typical day as starting with a 6 a.m. underground elevator ride that travels more than a mile underground. SNOLAB researchers share the elevator with miners on their way to work. “All the miners get very dirty, but all the SNOLAB people are clean, so the difference between them is stark. It’s very obvious who is the nerd underground and who the miners are,” says Marzec.
After arriving at the –6,800th floor, researchers walk more than a half mile from the cage shaft to the SNOLAB through underground dirt tunnels. When they reach the lab, they have to shower and change into threadless uniforms to prevent any microscopic threads from getting inside the sensitive detector. After air quality checks are completed, the researchers are free to begin their work on the detector.
When asked what it’s like to work more than a mile underground, Marzec comments that he got used to the strangeness after a few visits. “The first time, it feels very much like you’re underground because the pressure is very noticeable, and you feel exhausted at the end of the day.” Thankfully, Marzec and his colleagues don’t have to travel a mile underground every time they want to collect data from SNO+ since they can remotely collect and analyze the hundreds of terabytes of data generated by the detector.
As Marzec is in the final stages of preparing his Ph.D. thesis, he says he will miss his time working on SNO+. “It’s kind of monastic,” Marzec says about his time working at SNOLAB. “You go there and mediate on physics while you’re there. But it’s also kind of a social thing as well: There are a lot of people you know who are working on the same stuff.”
Klein and his group, including four graduate students and two post-docs, recently returned from a SNOLAB collaboration meeting, where upwards of 100 physicists met to present and discuss recent results and the upcoming plans for the next phase of the project. Klein is excited, and, admittedly, a little bit nervous, to see how everything comes together. “Putting in the liquid scintillator will change everything—there’s never been a detector being converted from a water-based detector to a scintillator detector. Here at Penn, for us, it’s big because we designed upgrades to the electronics to handle the fact that we will be getting data at a rate that’s about 100 times higher,” says Klein.
Despite the numerous technical and logistical challenges ahead, researchers are enthusiastic about the potential that SNO+ can bring to particle physics research. Other areas of study include learning how neutrinos change form, studying low-energy neutrinos to figure out why the Sun seems to have less “heavy” elements than astronomers expect, and measuring geoneutrinos to figure out why Earth is hotter than other nearby planets like Mars.
But for Klein, the prospect of finding a rare neutrinoless double beta decay event remains the most thrilling aspect of this research, which, if discovered, could turn the Standard Model of particle physics on its head. “After the question of what is dark energy and what is dark matter, the question of whether neutrinos are their own antiparticle is the most important question for particle physics to answer,” Klein says. “And if neutrinos are their own antiparticle, the simplest piece you can put into the equation [within the Standard Model] blows up: It doesn’t work, it’s mathematically inconsistent. And we don’t know how we would fix that. It is a completely experimental question, so that’s why we’re excited.”
Joshua Klein is the Edmund J. and Louise W. Kahn Professor and Graduate Chair in the Department of Physics & Astronomy in the School of Arts and Sciences.
Capital construction funds for the SNO+ experiment were provided by the Canada Foundation for Innovation (CFI) and matching partners. SNOLAB operations are supported by the CFI and the Province of Ontario Ministry of Research and Innovation, with underground access provided by Vale at the Creighton mine site.
The research was funded by the Department of Energy Office of Nuclear Physics, the National Science Foundation, and the Department of Energy National Nuclear Security Administration through the Nuclear Science and Security Consortium.
Homepage photo: Physics researchers here at Penn are responsible for the maintenance of the electronics inside the SNO+ detector. This involves designing new boards, testing existing components, and making regular trips to Canada for repairs.