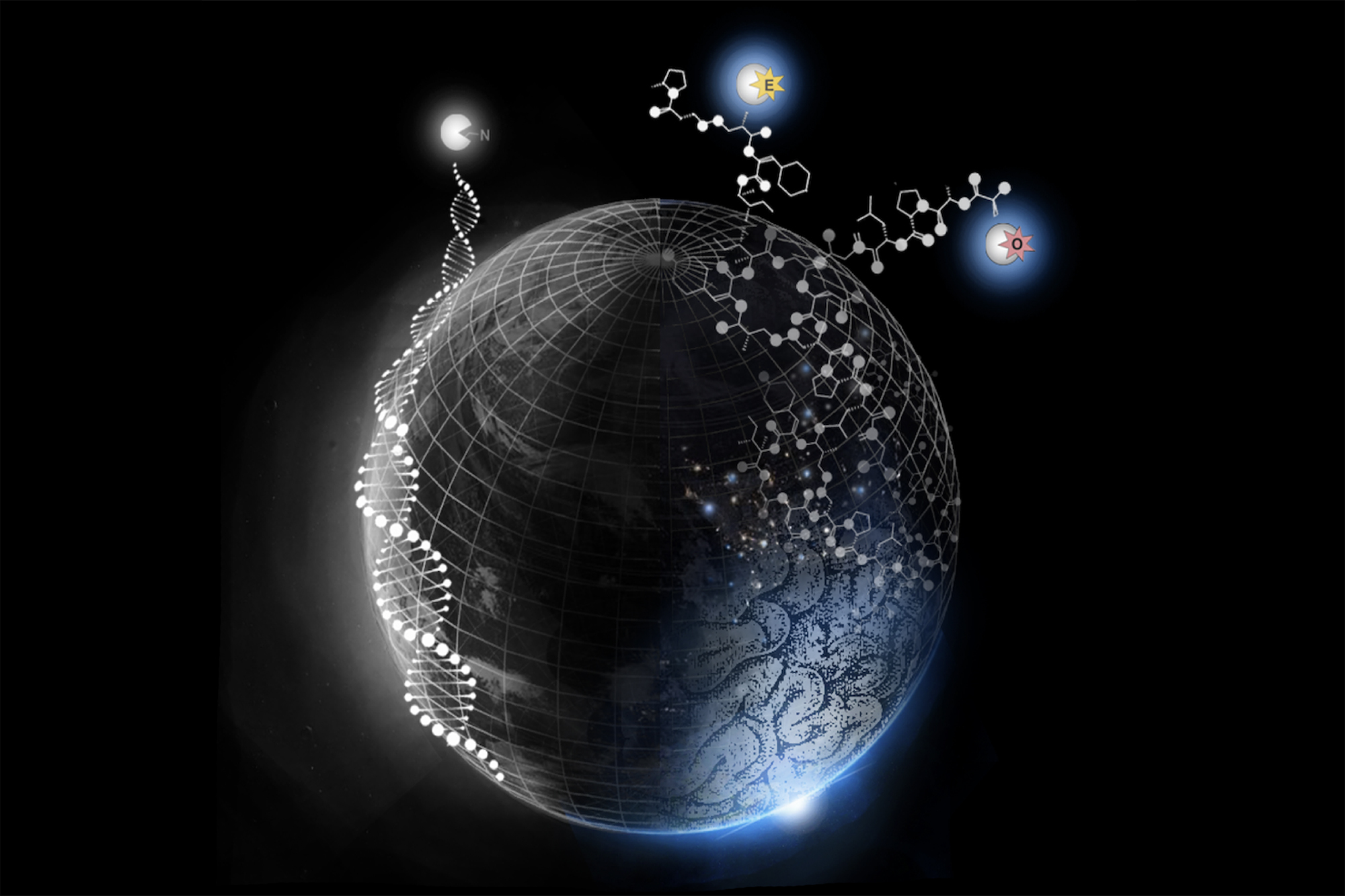
Researchers use synthesized small molecule tools, known as chemical probes, with druglike properties to identify specific types of proteins in order to find potential new drug leads. However, currently-available technologies are not able to access therapeutic targets that have metals, metabolites, or post-translational modifications.
Now, a study from the lab of Megan Matthews and colleagues suggests new ways to manipulate this class of disease targets that have so far remained “undruggable.” The findings were published in ACS Central Science and are featured on the publication’s September cover.
Scientists in the Matthews lab are chemical biologists who are multidisciplinary and collaborative. They have expertise in synthetic chemistry, enzymology, cell biology, and mass spectrometry-based chemical proteomics, which is commonly used to characterize small molecule-protein interactions and their effects on protein function. Using this approach, the researchers can globally profile and discover proteins that react with specific probes, understand what these proteins do, and inhibit the protein’s activities by novel mechanisms.
In this study, the researchers focused on mapping the chemical reactivity of an organohydrazine, –NHNH2, probe that mimics one of the first FDA-approved antidepressants, known as phenelzine, using a method called activity-based protein profiling (ABPP). Classical ABPP probes target a single type of amino acid that is nucleophilic, or electron-rich, whereas hydrazine probes are designed to capture enzyme cofactors and post-translational modifications that are electrophilic, or electron-poor.
“Hydrazines capture all kinds of really exciting targets by really interesting chemistry, so we’re using it as a launching point for enzyme-inhibitor discovery,” says Matthews, the principal investigator of this study. “We wanted to ask what are all the things that this pharmacophore can do proteome-wide, and because of mass spectrometry we can do this.”
After deploying their probe into two human cell lines, they showed that the probes react with targets from multiple enzyme classes that use a diverse range of cofactors; cofactors are different types of chemical machinery that help a protein do its function. Then, by mapping the locations of probe labelling on the proteins, the scientists demonstrated two modes of reactivity, called direct polar attack and oxidative activation/fragmentation, that rely on the versatile properties of hydrazine and its ability to capture different types of electron deficiency.
One of the biggest technical challenges, says postdoc and first author Zongtao “Tom” Lin, was identifying where and how the probe reacted with the proteins because it is not something that was easily predictable. “Our solution was to use isotopic hydrazine probes, replacing natural abundance nitrogen atoms 14N, with its ‘heavy’ counterpart 15N. This allowed us to see whether the hydrazine group was lost after reacting with the protein or not,” Lin says. “After that, we relied on a computational workflow to match the peptide fragmentation patterns and narrow down the sites of probe labeling. This combination of isotopic hydrazine probes and computational searches allowed us to achieve our goal.”
The team found that, although hydrazines are broadly reactive, they remain active-site directed and are blocked by other molecules that occupy a protein’s active site. “Because they are targeting functional chemistry, they are able to read out the functional state of many different enzyme classes. That’s pretty amazing because it approaches the single-probe Holy Grail to be able to profile any protein functionality that’s electron deficient,” Matthews says. “So now, in principle, we can develop selective molecules for targets found in this uncharted half of proteome that now is ‘druggable,’ and that’s really powerful and expansive.”
Next, the scientists will elaborate and tune these hydrazines to explore whether diversified nucleophiles have the same capacity as electrophiles to serve as potent, selective inhibitors of cofactor-dependent enzymes.
Matthews adds that because these methods are “disease agnostic,” there are unique opportunities to study activities that are dyregulated in patient samples and disease models. “Overall, we expect hydrazine probes to retain all the capabilities of classical ABPP probes, including the discovery of inhibitors and new mechanisms of action,” Matthews says. “In some cases, we hope to uncover some new biology, too.”
Zongtao Tom Lin is a postdoctoral student in the Department of Chemistry in the School of Arts & Sciences at the University of Pennsylvania. He is a postdoc researcher in the Matthews lab.
Megan L. Matthews is an assistant professor in the Department of Chemistry in the School of Arts & Sciences at the University of Pennsylvania.
The complete list of authors: Zongtao Tom Lin, Xie Wang, Katelyn Bustin, Kyosuke Shishikura, Nate McKnight, Mina Ahmadi, and Megan Matthews from Penn; Lin He from Zenagem; Radu Suciu and Erika Olson from the Scripps Research Institute; Kai Hu from the University of Massachusetts; Xian Han from St. Jude’s Children’s Research Hospital; and William Parsons from Oberlin College.
This research was supported by National Institutes of Health Grant NIDA 1DP1DA051620.