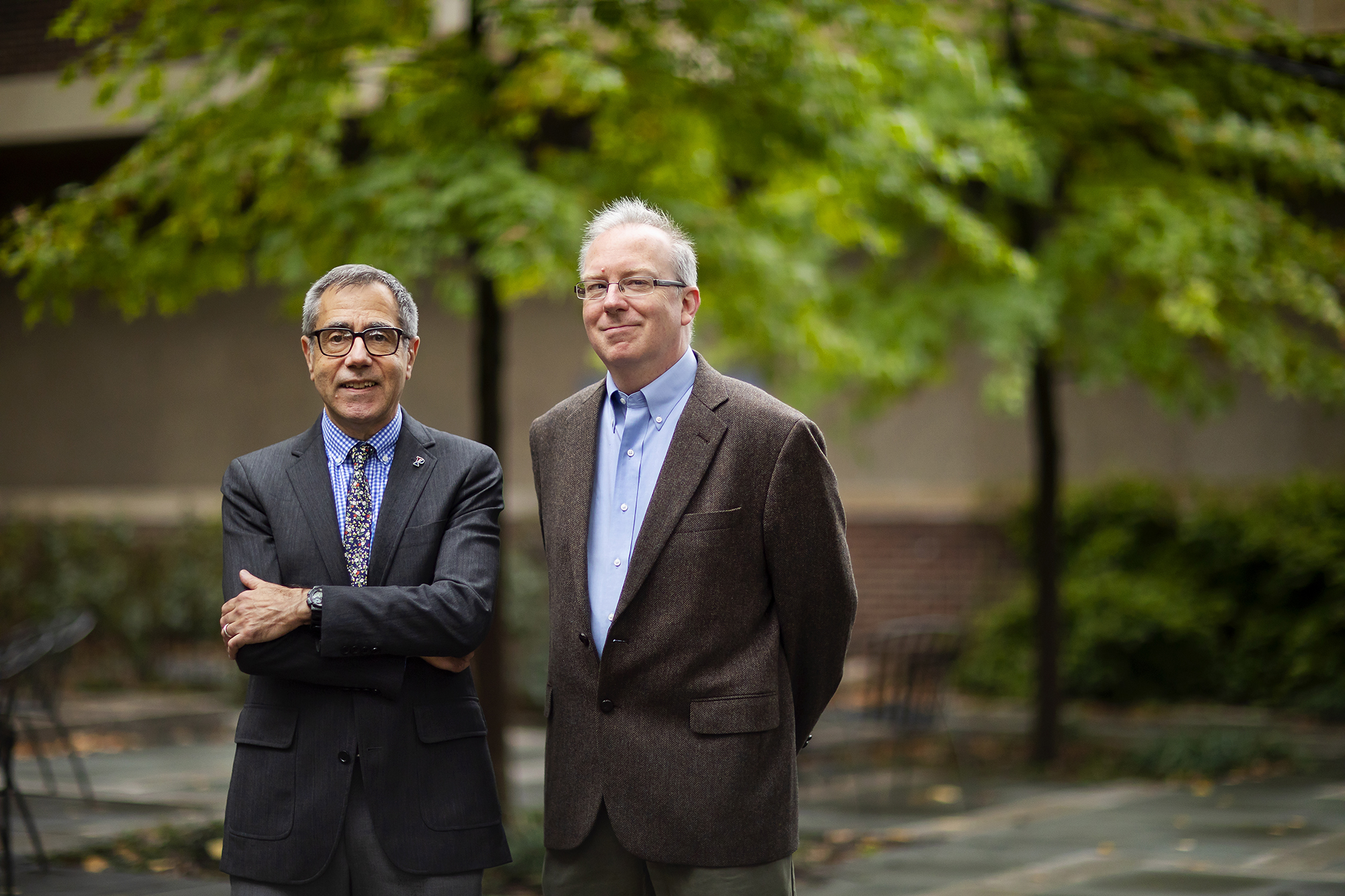
Two-and-a-half years ago, Charles Kane and Eugene Mele received the 2019 Breakthrough Prize in Fundamental Physics. The award recognized their theories that predicted the existence of topological insulators, a new class of material with the unique property of being an electrical insulator on the inside and having a surface that conducts electricity. Their work has had a profound impact on many areas of physics and provided new insights for understanding a material’s unique properties, with potential applications ranging from more efficient electronics to superfast quantum computers.
Now, as Kane and Mele mark 30 years of working together in the Department of Physics & Astronomy, the two continue to inspire a new generation of talented researchers. Here at Penn, this group includes both theorists and experimentalists who are delving deeper into these groundbreaking theories while working towards new applications and ideas.
Working next door and in parallel
While both are currently working from home, Kane and Mele have been office neighbors since 1991, where they share a “complementary” office and common area in the David Rittenhouse Laboratory. “When I arrived at Penn, I really benefited from Gene having his door open,” says Kane, who came to Penn 10 years after Mele’s arrival in 1981. “There were all these casual interactions that we would have, and even though we weren’t co-authoring papers together at that time, we were talking.”
One of their earliest topics of conversation was graphene. Long before atomically-thin carbon became a household name, Kane was wondering why graphene seemed to be on the “knife’s edge” between a conductor and an insulator. “I remember going into Gene’s office and telling him ‘I think graphene maybe should really be an insulator.’ That started this back and forth, and there was there was lots of synergy in that conversation,” says Kane.
Mele, who had been working on theories about graphene and carbon nanotubes at that time, argued that this effect would have been very weak in elements like carbon—but, as Kane retorted, they were still there. “The entire point of this discussion was whether or not something that was that weak is something that we really should be paying attention to, and certainly we did,” says Mele. “One of the great surprises to me was that, in a short period of time, people were able to take that idea and say, if you put it in a different class of material that have heavy atoms, this thing is not small. At that point, a rather interesting idea became an industry.”
These “animated” conversations during the summer of 2004 led to their two seminal papers “Quantum Spin Hall Effect in Graphene” and “Z2 Topological Order and the Quantum Spin Hall effect,” which introduced the application of topology to electronic band theory.
The two theorists, who now have co-authored more than 20 research papers together, describe their collaboration as both parallel and complementary—one that relies on their views from different areas of physics but that still allows them to understand each other’s perspectives.
“I personally gain a lot by having Charlie 30 feet away, even if I want to go in and just say what do you think of this. Charlie’s got a good nose for finding ideas that are not going to stand scrutiny, and at the end of the day, the stuff that goes out has to be right,” says Mele.
“The thing that I value about talking to Gene is I can bring something up with him and he’ll get what I’m talking about,” adds Kane. “We are critical of each other’s ideas but in a friendly and supportive way, and I think that’s something that really works.”
Bridging theory with experiments
After their seminal papers came three “waves” of experiments: Early work confirming that the predictions about graphene were true, expanded work showing that predictions about graphene were also found in another two dimensional material, and a later “explosion” of experiments highlighting how the phenomena could occur in three dimension. And while much of this work over the past 15 years was conducted elsewhere, Kane says that “it’s now coming back to Penn.”
Since joining Penn in July 2018, Liang Wu and his group have conducted research using advanced spectroscopy at unique frequency regimes, essential experiments for further evaluating and confirming topological properties in new materials. His group is particularly interested in the ways that light interacts with quantum materials, with recent studies including evidence of exotic particles in cobalt monosilicide and metal alloys that convert light into electric current.
With an eye toward applications ranging from quantum computing devices to more efficient optical-electronic devices, much of Wu’s work to date has been focused on experiments in the lab. Working in close collaboration with theorists, he says, is crucial for explaining their results and developing new ideas. “In our upcoming paper, I was expecting something else, but we saw something which I didn’t understand. I brought it to Charlie, and it turns out that it can be simply and elegantly explained by another theory. [Charlie and Gene] can always help you to understand some data, and it’s always very helpful,” says Wu.
Wu has been collaborating with Kane and Mele on a Materials Research Science and Engineering Centers (MRSEC) seed grant to study nonlinear optical properties of quantum materials and have produced four papers already. Wu is now working with Kane to probe new types of superconducting structures, ones that could be used in quantum computing applications. “We are using a new kind of nonlinear terahertz set up to probe the topological insulator and superconducting heterostructures to look for signatures of superconductivity that Charlie predicted ten years ago,” says Wu about this collaboration.
Bo Zhen, who has been at Penn since 2018, is applying ideas from topological electronics into a related field of topological photonics. Using a combination of experiments and theory, Zhen’s group is exploring how to fabricate materials so that they can have precise control over the flow of light, with recent studies highlighting this approach’s potential to reduce energy loss in optical fiber networks.
Because there are a number of fundamental differences in how optical and electronic systems work, new methods, materials, techniques, and even theories are needed to answer questions in this new field. “Photonics is learning from the electronics and now I think photonics has a chance of going on its own road,” says Zhen. “We’re bringing something unique and different, but the fundamentals are the same, so we constantly check with theorists. It is really a different problem now, but there is still a shared foundation.”
To that end, Zhen and Mele are collaborating on a MRSEC-funded project and have published three papers predicting new light-induced topological phases in certain types of nonlinear optical systems. The next challenge, says Zhen, will be demonstrating these effects in the lab and cataloging possible topological phases in the still relatively unexplored area of topological photonics. “It’s going to be interesting, from a theory point of view, to classify out all the possibilities and what possible topological phases there are when you shine light on them,” says Zhen.
The continuing role of theory
Martin Claassen, who joined Penn as an assistant professor last year, brings his expertise in developing theories that help describe the behavior of electrons in quantum materials and how their interactions with light can drive different properties.
“We have many properties that we desire to engineer for both fundamentally interesting reasons but also for practical applications,” he explains. “We’re trying to understand this intricate interplay of electrons, lattice dynamics, and mutual interactions that can give rise to interesting collective phenomena.”
Using a combination of mathematical theory and computer-based simulations, Claassen’s work focuses on out-of-equilibrium behaviors in quantum materials, where fundamentally new things can happen but that require new methods for understanding them. His goal is to predict ways that could help researchers find new properties or explain unexpected results. Here at Penn, he’s looking forward to the opportunity to work with both condensed matter theorists and experimentalists, and has been discussing with Wu about non-equilibrium problems in quantum materials.
“An experimental collaboration is a back-and-forth—We have common interests and oftentimes we try and predict something, they measure something, we try to understand the measurements, or maybe revise the prediction, and ultimately arrive at some interesting new physics,” Claassen says about his experience with theory and experiment collaborations. “The big questions are where to search for interesting new physics and how to understand experimental observations, so part of the work I do is modeling experimental observations and the other is trying to predict new phenomena.”
With an eye toward future applications
One example of an application for topological materials is for quantum computing devices and platforms. To this end, Marija Drndić and her lab are collaborating with Mele on a new type of two-dimensional material that has unique ferromagnetic properties, one that holds promise for developing quantum computing devices that would work at room temperature.
With so many different materials now up for grabs, says Drndić, part of the challenge is knowing where to start, which is where theory is essential. “Gene is able to predict out of all of these materials what is great to do. This is a 2D ferromagnetic material, which is new and promising, but then you can start dreaming of new phenomena you may see, devices you can build, that rely on new effects along the lines of work from Gene and others,” she says.
While her lab first sorts out how to work with this new material, future discussions about their results and working with Mele and other theorists will be the most interesting part of the collaboration, she says. “That’s the power of physics: The systems are simple enough that we have that luxury to have both experiment and theory. And the interplay is crucial—[theorists] give us ideas, but [experimentalists] also motivate theorists with a new material to think about the possibilities that could be there,” adds Drndić.
From novel ideas to future possibilities
To fully explore all of the potential of topological materials, continued collaboration between researchers working on theory and experiment will be key, says Kane. “The thing that is exciting about physics is you have matter that has these properties, and the question is what can you get it to do. Part of that is conceiving of things and part of it is actually making it happen, and the interplay between theorists and experimentalists is a crucial part of that,” he says.
“I think that theory has gone down this track where one is cataloging what kinds of special geometrical features one can expect to find, but really I think the grail here is to figure out what does that enable and what are the special properties you can actually see,” adds Mele.
Progress towards this “holy grail” is already underway here at Penn, with Wu and Kane studying topological phases in superconducting structures; Wu, Kane, and Mele studying nonlinear optical properties in quantum materials; and Zhen and Mele confirming their previous predictions on light-induced topological phases. Drndić’s collaboration with Mele on 2D materials is also ongoing, which opens up even more possibilities using bilayered or twisted single-layered version of this material, akin to what’s been done for graphene.
“One of the things that has evolved is that there are many, many more materials that people have at their disposal, so this is an area where I think is ripe for experimental progress to make these things come to life,” says Kane about the future of this field. “It’s an amazing thing what the experimentalists are able to do.”
But even as methods, materials, and experiments improve, there will continue to be a strong need for solid theories to underpin and explain new results. This is especially true for topological photonics, a field that’s now poised to do something different but that still relies on the foundational work by Kane and Mele. “From the beginning, it’s been the theory leading the experiments for a very long time,” says Zhen. “We definitely benefit greatly from everything that they done.”
For Drndić, it’s exciting to see how things have evolved, especially as it relates to quantum computing applications, a once “pie in the sky” idea that’s now becoming real, she says. This is thanks in part to Kane and Mele’s “curated” and mathematical approaches to addressing problems in physics. “What is great is that they were able to predict novel phenomena starting from basic physics concepts that ended up being very powerful,” she says. “It’s cool that it still happens in physics, and great that the theory and the mathematics works.”
And while Claassen is still new to Penn, his theoretical background provides numerous opportunities for coupling innovative theories with new experiments and potential future applications. There are many open questions, he adds, and part of the future of this field will likely include variations on and expansions of Kane and Mele’s groundbreaking theories.
“There’s open questions in topological phases that promise a rich playground to generate new phenomena, which all lead back to Charlie and Gene’s pioneering works and their profound results on topology in condensed matter have really been one of the guiding inspirations throughout my research,” he says. “For some of these problems, we’re just at the beginning of understanding their depth and what is possible.”
Martin Claassen is an assistant professor in the Department of Physics and Astronomy in the School of Arts & Sciences at the University of Pennsylvania.
Marija Drndić is the Fay R. and Eugene L. Langberg Professor of Physics in the Department of Physics and Astronomy in Penn’s School of Arts & Sciences.
Charlie Kane is the Christopher H. Browne Distinguished Professor of Physics in the Department of Physics and Astronomy in Penn’s School of Arts & Sciences.
Eugene Mele is the Christopher H. Browne Distinguished Professor of Physics in the Department of Physics and Astronomy in Penn’s School of Arts & Sciences.
Liang Wu is an assistant professor in the Department of Physics and Astronomy in Penn’s School of Arts & Sciences.
Bo Zhen is an assistant professor in the Department of Physics and Astronomy in Penn’s School of Arts & Sciences.