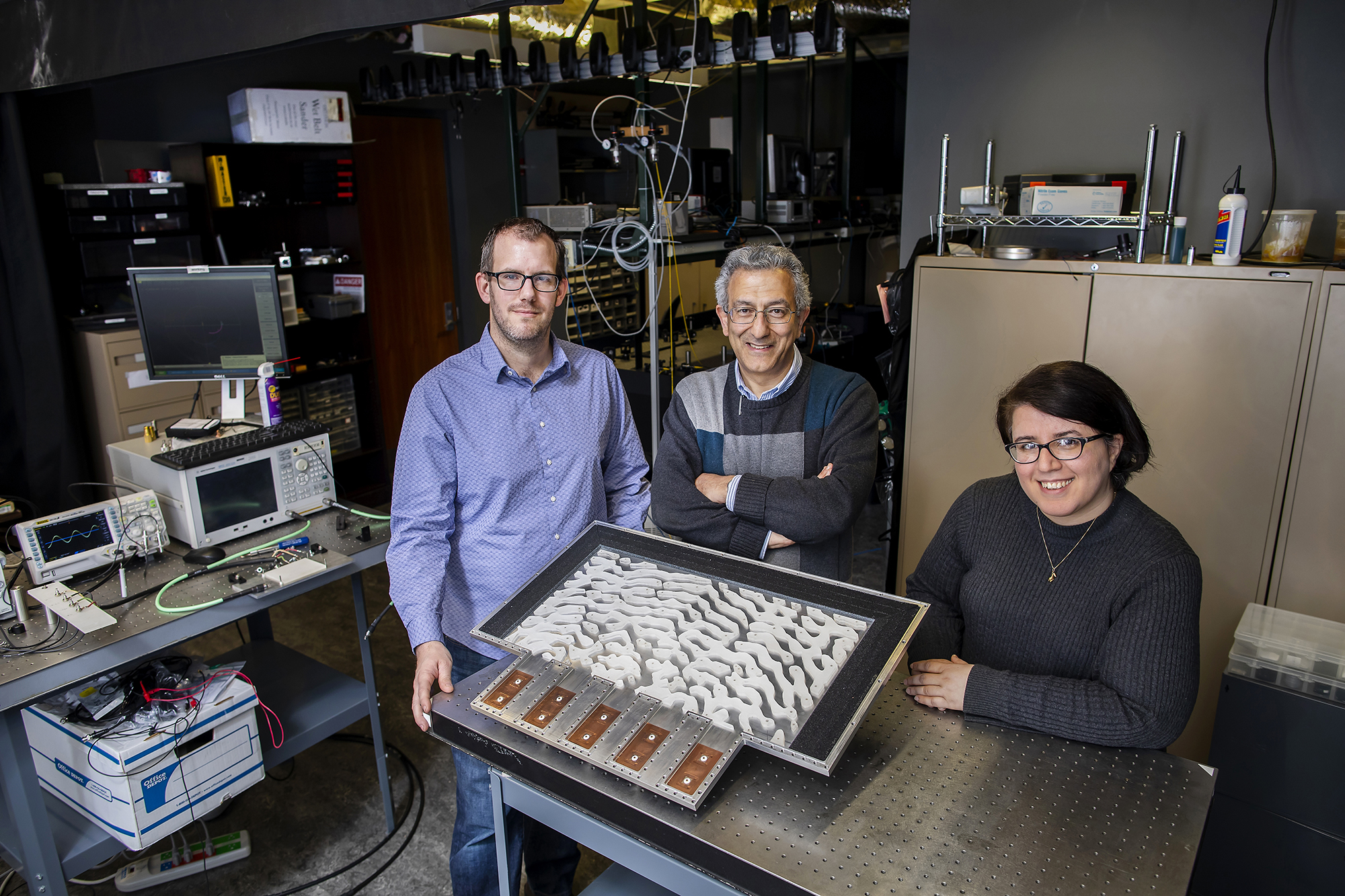
The field of metamaterials involves designing complicated, composite structures, some of which can manipulate electromagnetic waves in ways that are impossible in naturally occurring materials.
For Nader Engheta of the School of Engineering and Applied Science, one of the loftier goals in this field has been to design metamaterials that can solve equations. This “photonic calculus” would work by encoding parameters into the properties of an incoming electromagnetic wave and sending it through a metamaterial device; once inside, the device’s unique structure would manipulate the wave in such a way that it would exit encoded with the solution to a pre-set integral equation for that arbitrary input.
In a paper published in Science, Engheta and his team demonstrated such a device for the first time.
Their proof-of-concept experiment was conducted with microwaves, as the long wavelengths allowed for an easier-to-construct macro-scale device. The principles behind their findings, however, can be scaled down to light waves, eventually fitting onto a microchip.
Such metamaterial devices would function as analog computers that operate with light, rather than electricity. They could solve integral equations—ubiquitous problems in every branch of science and engineering—orders of magnitude faster than their digital counterparts, while using less power.
Engheta, the H. Nedwill Ramsey Professor in the Department of Electrical and Systems Engineering, conducted the study along with lab members Nasim Mohammadi Estakhri and Brian Edwards.
This approach has its roots in analog computing. The first analog computers solved mathematical problems using physical elements, such as slide-rules and sets of gears, that were manipulated in precise ways to arrive at a solution. In the mid-20th century, electronic analog computers replaced the mechanical ones, with series of resistors, capacitors, inductors, and amplifiers replacing their predecessors’ clockworks.
Such computers were state-of-the-art, as they could solve large tables of information all at once, but were limited to the class of problems they were pre-designed to handle. The advent of reconfigurable, programmable digital computers, starting with ENIAC, constructed at Penn in 1945, made them obsolete.
As the field of metamaterials developed, Engheta and his team devised a way of bringing the concepts behind analog computing into the 21st century. Publishing a theoretical outline for “photonic calculus” in Science in 2014, they showed how a carefully designed metamaterial could perform mathematical operations on the profile of a wave passing thought it, such as finding its first or second derivative.
Now, Engheta and his team have performed physical experiments validating this theory and expanding it to solve equations.
“Our device contains a block of dielectric material that has a very specific distribution of air holes,” Engheta says. “Our team likes to call it ‘Swiss cheese.’”
The Swiss cheese material is a kind of polystyrene plastic; its intricate shape is carved by a CNC milling machine.
“Controlling the interactions of electromagnetic waves with this Swiss cheese metastructure is the key to solving the equation,” Estakhri says. “Once the system is properly assembled, what you get out of the system is the solution to an integral equation.”
“This structure,” Edwards adds, “was calculated through a computational process known as ‘inverse design,’ which can be used to find shapes that no human would think of trying.”
The pattern of hollow regions in the Swiss cheese is predetermined to solve an integral equation with a given “kernel,” the part of the equation that describes the relationship between two variables. This general class of such integral equations, known as “Fredholm integral equations of the second kind,” is a common way of describing different physical phenomena in a variety of scientific fields. The pre-set equation can be solved for any arbitrary inputs, which are represented by the phases and magnitudes of the waves that are introduced into the device.
“For example," Engheta says, "if you were trying to plan the acoustics of a concert hall, you could write an integral equation where the inputs represent the sources of the sound, such as the position of speakers or instruments, as well as how loudly they play. Other parts of the equation would represent the geometry of the room and the material its walls are made of. Solving that equation would give you the volume at different points in the concert hall.”
In the integral equation that describes the relationship between sound sources, room shape and the volume at specific locations, the features of the room — the shape and material properties of its walls — can be represented by the equation’s kernel. This is the part the Penn Engineering researchers are able to represent in a physical way, through the precise arrangement of air holes in their metamaterial Swiss cheese.
“Our system allows you to change the inputs that represent the locations of the sound sources by changing the properties of the wave you send into the system,” Engheta says, “but if you want to change the shape of the room, for example, you will have to make a new kernel.”
The researchers conducted their experiment with microwaves; as such, their device was roughly two square feet, or about eight wavelengths wide and four wavelengths long.
“Even at this proof-of-concept stage, our device is extremely fast compared to electronics,” Engheta says. “With microwaves, our analysis has shown that a solution can be obtained in hundreds of nanoseconds, and once we take it to optics the speed would be in picoseconds.”
Scaling down the concept to the scale where it could operate on light waves and be placed on a microchip would not only make them more practical for computing, it would open the doors to other technologies that would enable them to be more like the multipurpose digital computers that first made analog computing obsolete decades ago.
“We could use the technology behind rewritable CDs to make new Swiss cheese patterns as they’re needed,” Engheta says. “Some day you may be able to print your own reconfigurable analog computer at home!”
Nader Engheta is the H. Nedwill Ramsey Professor in the Department of Electrical and Systems Engineering at the University of Pennsylvania's School of Engineering and Applied Science.
The research was supported by the Basic Research Office of the Assistant Secretary of Defense for Research and Engineering through its Vannevar Bush Faculty Fellowship program and by the Office of Naval Research through Grant N00014-16-1-2029.