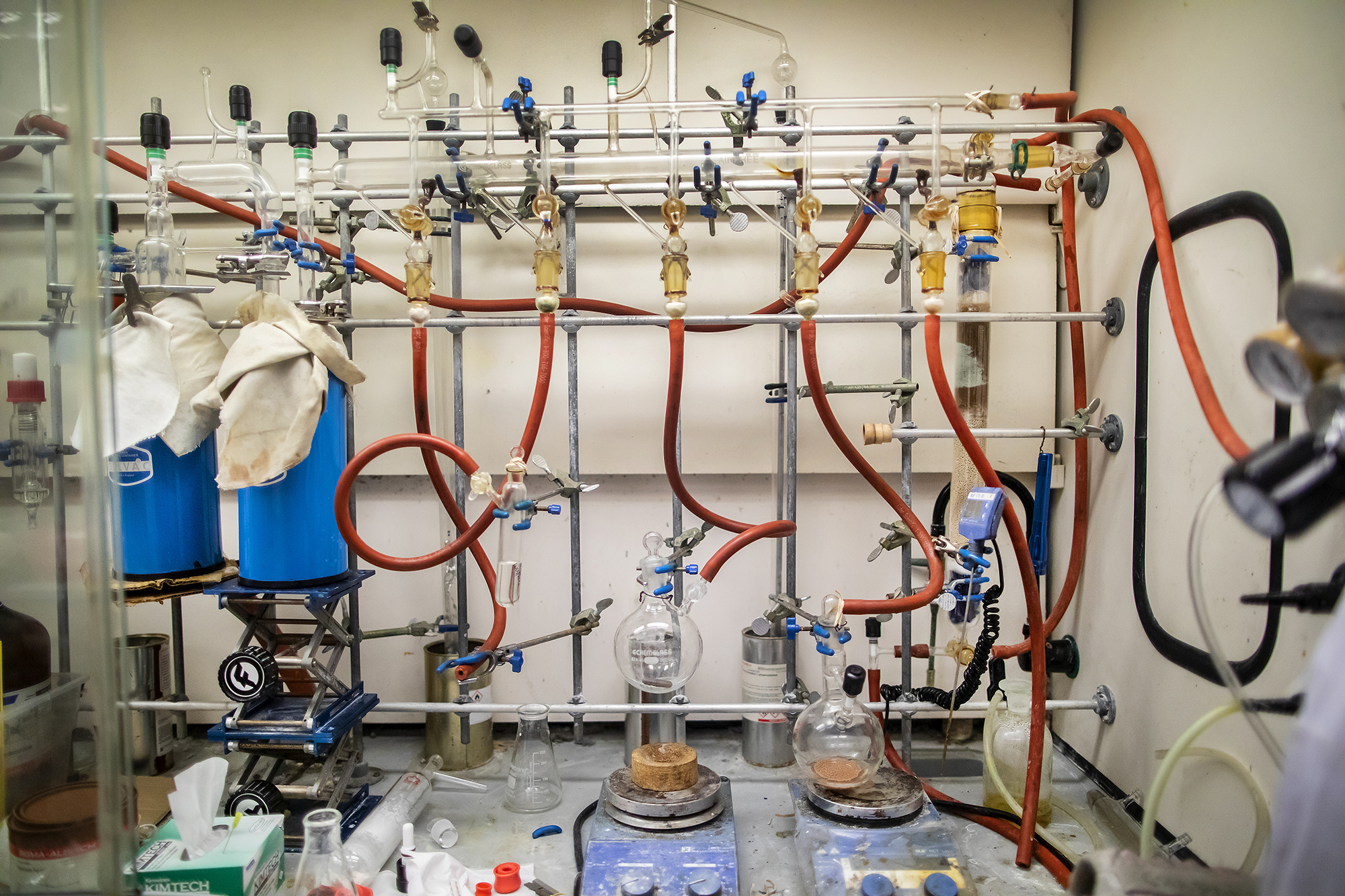
Whether it’s making batteries more efficient or finding new ways to reuse natural resources, energy research is a multifaceted area of study that requires both solid science and a creative approach to solving problems.
In the lab of Neil Tomson, researchers are asking fundamental questions related to the energy crisis in the hopes of developing more efficient ways to do chemical reactions. Now, as part of a three-part phased reopening of research activities, his group members are back in the lab reviving “old school” techniques to tackle problems in inorganic chemistry using creativity and innovation.
Catalysts in industry
Tomson and his group focus on catalysts, a key factor in how quickly and efficiently more than 90% of industrial reactions occur. “Catalysis is inherently an energy problem. It’s finding ways to do chemical transformations in a way that uses less energy,” says Tomson.
There are two main types of catalysts, homogeneous and heterogeneous, each with different structures and ways that they support chemical reactions. Heterogeneous catalysts are of interest because they are easier to separate from the products they create and are also much more stable than homogeneous systems, but they have been notoriously difficult to study and synthesize in the lab.
Since both types of catalysts rely on transition metals, the Tomson lab is looking for ways to create homogeneous catalysts that act more like heterogeneous catalysts and are using transition metals as a starting point. Part of their work involves creating new “scaffoldings,” in this case organic ligands, that hold the metal in a place where it can be the most efficient. “The metal does all the interesting chemistry, but it’s being controlled by the ligand that’s bound to it,” says Tomson. “It’s a challenge to find ligands that place metal ions in new and unusual geometries, but those new geometries are needed for accessing new reactivity."
To address this challenge, the lab is looking for ways to mimic the chemistry that happens on the surfaces of transition metals by creating large, multiple-ion complexes with scaffolding that holds the ions in just the right place. Using this same strategy of looking at the key features of catalytic systems, the group is also studying how electrons move within the active sites of enzymes.
Their goal is to delve into the fundamentals of catalysis so they can come up with new and creative ways to make the chemical reactions that rely on them more efficient. “We try and capture features that people have thought about for a long time but haven’t had a great way of translating into compounds,” Tomson says.
Metal-surface mimics and enzyme electrostatics
Graduate student Qiuran Wang creates organic compound scaffolds that hold groups of metal ions together to mimic a metallic surface and compares their structures to single-ion systems. His goal is to figure out what specific characteristics are key so they can be engineered into new catalysts. “This project mimics the whole metal surface,” says Wang. “Aside from the individual metal active sites, they have a sea of electrons surrounding them, and we are using redox active ligands to store electrons so that the metal center is supported by those ligands.”
Wang recently created a series of compounds that mimicked the interactions between metals on metallic surfaces, and more recent research has focused on the ability of this ligand system to adapt to the needs of the metal ions in its core. “These compounds do a great job of mimicking both the change in geometry and the change in electronic structure that are known to occur on metallic surfaces,” says Tomson.
On the other side of the lab, graduate student Alex Weberg designs new molecules for studying how chemical reactivity is impacted by electric fields. One way that enzymes catalyze reactions is by placing a positive (or negative) charge near a reaction site in a way that alters its electron density. “We’re looking at putting charged residues into the outer sphere of our complexes to affect how small molecules bind or react when they interact with a metal,” says Weberg.
Having more in-depth knowledge of electronic structures will make it possible to design new catalysts that can do chemical reactions in a more controlled manner. “We are generating complexes that create an electrostatic field near where a small molecule binds, and we use the position of those charged residues as a way to induce the chemistry we’re going after,” says Tomson.
Classic chemistry with a modern twist
Members of the Tomson lab work with very reactive chemicals, ones that are no longer usable if they come into contact with air or water. The group does much of their work on Schlenk lines, a system of vacuum manifolds that keep their chemicals isolated from the atmosphere. Relying on this “old school” technique is challenging, as a simple error or even a drop in pressure can lead to multiple days of work being lost.
But, despite the challenges of using these techniques, Schlenk lines are still standard practice and remain a powerful tool for a chemist’s skillset. “It’s a huge advantage because there are some reactions you cannot do in the glove box,” says Weberg, referring to another method for working with reactive chemicals. “If I was somebody that had never learned Schlenk techniques, the easier thing would be to just not run that reaction.”
Because Wang works with materials that have complex electronic structures, he also relies on new analytical and computational approaches to learn more about the chemicals he creates. The compounds “have very interesting reactivities. I always get unexpected results, which is nice, but it’s hard to find out what’s going on, so I use characterization,” he says.
To learn more about the compounds they create, Tomson lab members like Wang rely on modern tools such as NMR spectroscopy, which allows a detailed look at specific parts of a single molecule, X-ray crystallography to study the structure of different compounds, and a superconducting quantum interference device (SQUID for short) to look at the number of unpaired electrons and the overall electronic structure of their compounds.
How to think about thinking
In his first semester general chemistry course, Tomson began introducing elements of metacognition, or "thinking about thinking," by having students explain complex scientific topics to others. “The process of explaining something to somebody else helps sharpen your focus and your understanding, and that’s an important aspect of metacognition,” he says.
Thanks to Tomson’s NSF CAREER award, the lab members will also be spearheading a series of “role model” videos for high school students. The videos will introduce energy science concepts through peer mentors while also getting graduate students active in metacognition activities. “Graduate students, in designing the videos and thinking about how to lead a discussion, consider their explanations and understanding of the material in a way that they are not often responsible for in their education,” says Tomson.
Weberg, who created the well-received pilot video, says the long-term goal is to get other labs at Penn involved with the outreach. “We’re becoming experts in these fields, and it’s hard to explain in a very simple way through visual and audio cues,” says Weberg. “You are communicating to students that wouldn’t otherwise see energy research.”
The future of efficient chemistry
After a long hiatus from lab work, the Tomson group members are now back to collecting data, working in small teams, and coming to campus in shifts. “This makes it hard to build momentum in research, but the students have been resilient,” says Tomson. “It has been exciting for all of us to have data coming in again.”
Tomson adds that the shutdown period was still a productive time for his group. They published five papers and have another two papers ready to be submitted soon. It’s been difficult to not go to the lab, says Tomson, but he is proud of what his group has achieved despite the circumstances. “I feel fortunate to have a group of students who work hard, stay engaged, and keep a level head through challenges and uncertainties,” he adds.
As his group starts picking up momentum, Tomson hopes to start making steady progress on developing new types of catalysts once more. “I don’t think it’s practical to say we’re going to be solving the energy problem in the next five to 10 years, but we want to inform the way people think about catalysts and different ways that you’ll be able to generate compounds over the long term,” he says.
Thanks to his knowledge of fundamental chemistry techniques and multiple opportunities to develop his communication skills, Wang is exploring opportunities in both industry and academia and is confident that he can find interesting and rewarding work in either sector. “I’m ready to get any challenge from anywhere,” he says.
After spending a year as a Vagelos Institute for Energy Science and Technology graduate fellow, Weberg met students and faculty working on energy-related problems in physics, engineering, and materials science and is now interested in a career in science policy. He continues to be impressed with the range of energy science research across campus. “Every aspect of technology can be improved, and people are doing it in really creative ways,” he says.
Interdisciplinary perspectives will continue to be crucial to the future of energy science along with skills in communication and metacognition. Thanks to their work both inside and outside the lab, researchers in the Tomson lab will be poised to do both.
“We need an all-hands approach to finding solutions,” says Tomson. “The energy challenge is going to be solved by people who do interesting chemistry that will also be appealing to industry. That means the chemists can talk to engineers and policy people to come to a solution that satisfies a lot of different demands.”
Ongoing research in the Tomson lab is supported by the National Institute of General Medical Sciences, School of Arts & Sciences, Department of Chemistry, and Vagelos Institute for Energy Science and Technology.
Neil C. Tomson is an assistant professor in the Department of Chemistry in the School of Arts & Sciences at the University of Pennsylvania.