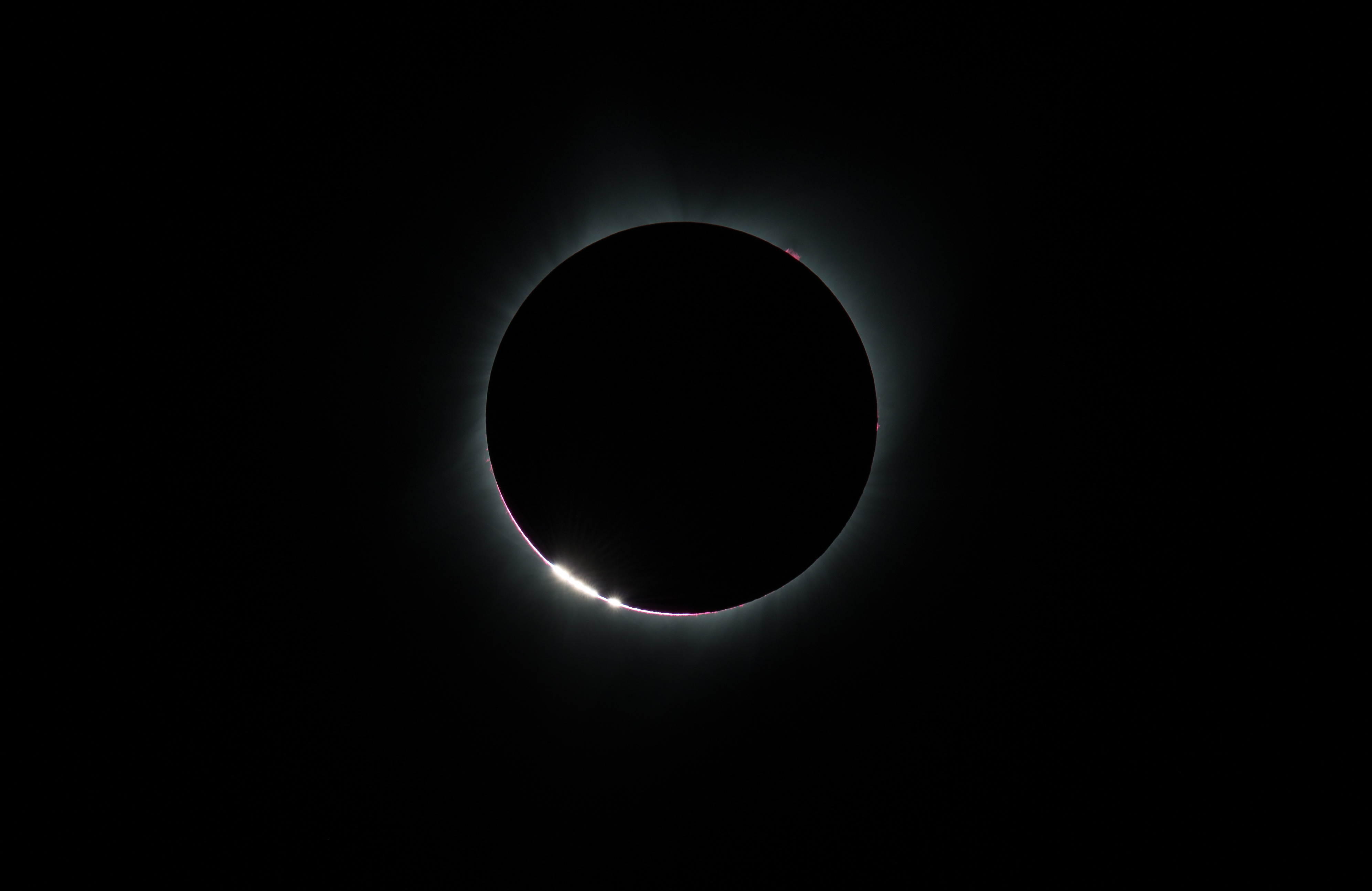
On April 8, large swathes of the United States will temporarily experience day turning into night as the moon passes in front of the sun with its shadow causing a total solar eclipse. The shadow will enter from Texas, travel through Oklahoma, Arkansas, Missouri, Illinois, Indiana, Ohio, Pennsylvania, New York, Vermont, and New Hampshire, finally exiting via Maine.
Penn Today met with astronomers Gary Bernstein and Bhuvnesh Jain of the School of Arts & Sciences to learn more about the mechanics of an eclipse, the coming one’s significance, and how eclipses have historically informed scientists understanding of the nature of the universe.
What is an eclipse?
“Simply put,” Jain says, “it’s when the moon blocks the sun.” He adds that eclipses involve an interesting coincidence: Although the sun is much larger than the moon, it is also much further away, which makes them appear to be very similar in size from Earth. This similarity in apparent size is critical for eclipses, where the moon can cover the sun completely or partially, depending on their relative positions and distances at the time.
Bernstein says that “the apparent similarity in size between the sun and the moon, is essentially an accident of current celestial mechanics. This unique configuration wasn’t the same a billion years ago and will change in the distant future because the moon’s gradually getting farther and farther away from Earth. As a result, in the distant future, the moon will be too small to completely cover the sun. Luckily that day is hundreds of million years away,” he says.
Total vs. partial eclipses
Bernstein notes that during a total solar eclipse the observer is in the moon’s full shadow, the umbra, which is only about 100 miles wide. This shadow traverses a path across Earth and being within this path allows observers to experience the total eclipse. “On the other hand, if you’re outside this narrow path, you’ll be in the penumbra, where the sun is only partially covered by the moon, resulting in a partial eclipse,” he says.
Bernstein emphasizes that a partial eclipse, even if it covers a large percentage of the sun, doesn’t compare to the awe-inspiring experience of a total eclipse. The difference in experience between a 98% and a 100% eclipse is significant; a nearly complete eclipse is not nearly as noticeable as when the eclipse is total, comparing it a cloud passing by. “So, unfortunately, being in 98% eclipse is only about 1% as interesting as being 100%,” he jokes. “Totality brings about dramatic changes in the environment, such as darkness and a noticeable drop in temperature, which doesn’t happen during a partial eclipse.”
How eclipses are predicted
Bernstein says that even in ancient times, such as during the era of the Greeks, people had begun to recognize patterns that suggested certain times of the year when eclipses might occur, although they couldn’t be certain of an eclipse’s occurrence. This alignment typically happens approximately every six months during what’s known as an eclipse season, specifically when the new moon phase coincides with the moon’s twice-monthly crossings of Earth’s orbital plane.
"Due to the moon’s orbit being tilted about 5 degrees relative to the Earth’s orbit, its shadow usually misses the Earth, making solar eclipses less frequent than monthly new moons,” Bernstein says. “Eclipses can only happen during eclipse seasons, resulting in two solar eclipses in a typical year, with most being only partial.”
Total eclipses are rarer because they require a more precise alignment between the centers of the sun and moon, and also because the moon’s apparent size in the sky is sometimes too small to fully cover the sun, Bernstein says. Since the moon’s orbit around Earth is slightly elliptical, as is Earth’s orbit around the sun, the apparent sizes of the sun and moon vary, affecting the type of eclipse that occurs.
The prediction of eclipses became more accurate after the publication of Newton’s laws of motion, as these laws allowed for a better understanding of celestial mechanics.
In contemporary times, Jain says, the positions of the earth, sun, and moon are known with remarkable precision, down to a few centimeters, thanks to extensive tracking of their movements across the sky and to monitoring by spacecraft. “This precise knowledge lets us astronomers predict eclipses with great accuracy, removing any doubt about when and where they will happen,” he says.
What eclipses can tell us about the universe
“In 1919, a total solar eclipse prompted astronomers Frank Watson Dyson and Arthur Stanley Eddington to set out on two expeditions to measure the gravitational deflection of starlight near the sun. This was the first observed instance of the bending of starlight around the sun, a phenomenon predicted precisely by Einstein,” Jain says. “The measurement of this deflection, which we call gravitational lensing, was one of the most significant moments in physics. It showed that massive objects like the sun could warp the fabric of space-time, bending the path of light passing near them.”
Bernstein notes the significance of the 1919 eclipse referring to a famous New York Times headline, “EINSTEIN EXPOUNDS HIS NEW THEORY,” followed by “It Discards Absolute Time and Space, Recognizing Them Only as Related to Moving Systems.” He says the news celebrated the observational confirmation of Einstein’s predictions, highlighting the impact of the event on both the scientific community and the broader public, leading to widespread acceptance of his theory and his rise to fame.
Bernstein says that “phenomena like gravitational lensing and the solar corona are only visible during total eclipses.” He explains that the corona is the outermost layer of the sun’s atmosphere, characterized by its hot, ionized gas exchanges that extend millions of miles into space, creating a halo-like or crown-like effect. “These two things are always there but are overwhelmed by the sun’s brightness. Total eclipses offer a rare opportunity to observe these phenomena directly from earth,” he says.
Nearly a century after the Eddington experiment, Jain says, the Kepler space telescope used a similar concept of shadow blocking for a groundbreaking purpose: the discovery of exoplanets. “Unlike the dramatic shadow of a solar eclipse, these distant planets obscure a minuscule fraction, often less than 1%, of their host star’s light,” he says. “Yet, Kepler’s precise measurements have unveiled a treasure trove of information about these exoplanets, leading to the astonishing realization that most stars in the Milky Way are accompanied by planets, expanding our understanding of the universe’s complexity.”
Where to see this eclipse
Bernstein is headed to Texas with friends and family to view the eclipse, “I’m really hoping for clear skies so they don’t blame me for dragging them down there if the eclipse is blocked by clouds,” he jokes. Jain remarks that he is envious of his neighbor who intends to ski to Whiteface Mountain in Lake Placid to experience the eclipse from a high vantage point before the expected time of the eclipse at 3 p.m.
“Being at a high elevation on a mountain can provide a clearer and more unobstructed view of the sky, offering a more immersive experience of the eclipse,” Jain says. “Also, from such a vantage point, it’s possible to see the approaching shadow of the moon over the landscape, before it envelops the mountaintop, adding to the excitement of eclipse viewing.”
“Whether you view the eclipse from a mountain top, on the flat expanse of the plains, or even a partial eclipse somewhere in Philly,” says Jain, “remember that it is never safe to view the exposed disk of the sun without proper eye protection. NASA has some useful guides for viewing eclipses safely that may be helpful.”
Gary Bernstein is the Reese W. Flower Professor of Astronomy and Astrophysics Professor in the Department of Physics and Astronomy in Penn’s School of Arts & Sciences.
Bhuvnesh Jain is the Walter H. and Leonore C. Annenberg Professor in the Natural Sciences and the co-director of the Center for Particle Cosmology in the Department of Physics and Astronomy in Penn’s School of Arts & Sciences.