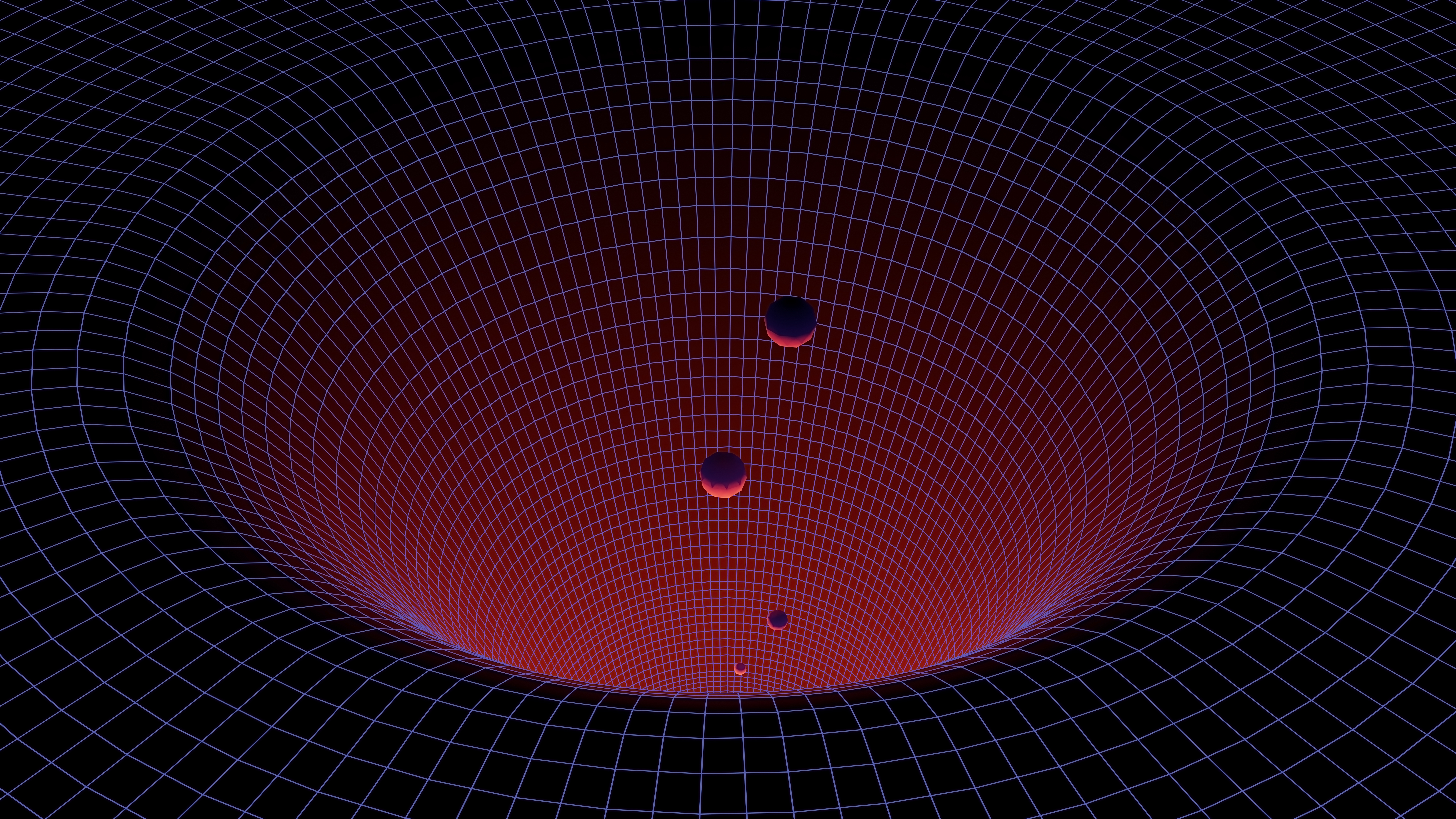
A recent Nature publication continues to generate headlines over its findings that scientists from the California Institute of Technology developed a model of a traversable wormhole on the Google Sycamore quantum processing system.
Penn Today spoke with physicists Vijay Balasubramanian and Jonathan Heckman from the Department of Physics and Astronomy in the School of Arts & Sciences to better understand the implications of this work. The two explained a few key concepts and shared their thoughts and opinions on some of the main take aways.
Can you explain what these researchers did?
Balasubramanian: These Caltech researchers were able to represent wormhole-like conditions on a quantum computer.
They’ve used a quantum computer to construct a simple version of a model often used to understand strongly correlated materials, that is materials in which the basic components strongly influence the behavior of each other. This is the so-called SYK model, named after the condensed matter physicists who initially proposed it, Subir Sachdev and Jinwu Ye, along with Alexei Kitaev, who later modified it. Famously, this SYK model has an equivalent description in terms of a certain theory of gravity in a universe with just one spatial dimension. In the Nature paper, the researchers built a quantum system that realized a stripped-down version of the SYK model and demonstrated characteristic dynamics that would be associated with a traversable wormhole in the alternative, and equivalent, gravitational description.
So, they haven’t made an actual wormhole?
Balasubramanian: No, they haven’t created a wormhole, or a shortcut connection between two distant points in space. But what they’ve been able to achieve here is still very impressive and a good step forward for quantum computing.
What do you mean by quantum computing, and why was it needed for this experiment?
Heckman: Well, as opposed to an ordinary computer system that uses binary bits corresponding to 0’s and 1’s in receiving, processing, storing, and communicating information, a quantum system has a ‘superposition’ of 0’s and 1’s, meaning its bits, known as qubits, are able to simultaneously exist as a zero or one.
So, the hope and promise of a quantum computer is that you could do computations that you would not be able to do on a classical machine, if you have enough qubits.
And from my understanding, it seems these researchers were motivated by what’s known as anti-de Sitter/conformal field theory (AdS/CFT) correspondence, which much like the SYK model is useful for studying phenomena in systems whose components interact strongly with each other. But the AdS/CFT correspondence is particularly useful for studying equivalencies between two different types of physical theories.
Balasubramanian: The AdS/CFT correspondence can be likened to expressing an idea in one language, then using a dictionary and grammar book to convey that same idea in a completely different set of sounds and grammatical practices associated to another language.
In a bit more detail, the AdS/CFT correspondence gives a dictionary and set of physical rules for translating phenomena in certain kinds of higher-dimensional gravitating universes (so-called Anti-de Sitter Spaces) to phenomena in other lower-dimensional systems (so-called Conformal Field Theories) that don’t experience gravity. This correspondence actually has roots in a well-established notion in physics dating back to the late 19th century, which we refer to as a duality, but the new incarnation of duality is one is one of the most important discoveries in physics in the last quarter century.
It seems initially incredible, perhaps impossible, that theories in different numbers of dimensions could be equivalent. After all, you would think dimension is kind of fundamental to the nature of physics; normally you feel that dimensions of space are kind of like a stage, that you can go backwards, forwards, left, right, up, or down in. But it’s turned out that we can construct examples where, for instance, you have some interaction happening in a three-dimensional theory that doesn’t contain gravity and you can show that it is equivalent to some other process in a four-dimensional theory with gravity.
Heckman: And based on that, the Caltech researchers had an expectation that they could use the quantum processor to teleport, or translate, pieces of quantum information from one region to another without losing any fidelity in the signal. Via the AdS/CFT duality, in the equivalent gravitational description with an extra dimension, you would say that the signal went through a wormhole. But in the actual machine they used there isn’t one.
In fact, there’s a version of this Nature paper that you could have written that makes absolutely no reference to quantum gravity or the AdS/CFT model.
How so? Wouldn’t that negate the point of studying wormhole-like environments for parsing information?
Heckman: Basically, the idea here is that gravity encodes information via a sort of hologram. In an actual hologram, you can have a two-dimensional system (like an image etched on a surface) that can be used to fully encode the original three-dimensional shape. Similarly, in the AdS/CFT duality, information of a higher dimensional gravitational system is encoded in a lower-dimensional system.
But in terms of what they did, it gets tricky here because they motivated the experiment based on using some special considerations of gravity: particularly, this idea of a wormhole configuration of gravity. And to get a wormhole, you’d have to create a bridge between two black holes. The black holes in question don’t exist in our world. Rather, they are present in an alternative, ‘dual,’ description of their quantum computing system in terms of a theory of gravity in a different number of dimensions.
So, in a sense, they used this ‘dual’ notion of gravity to imagine a traversable bridge, or wormhole, connecting two different quantum mechanical systems, and translated this back into equivalent phenomena in the actual system they built on their quantum computer.
Much of the media coverage of this Nature paper suggests that the researchers have created a wormhole. What do you make of these claims?
Heckman: Insinuating that there is an actual wormhole traversal happening in our world is quite misleading. The authors of the article and the press covering it are engaging in very irresponsible representations of the work.
This particular model of gravity works best when the number of qubits is really large, as in, it approaches infinity, which isn’t possible right now, so the researchers address this by using a deep learning network to help them build a small enough quantum system that retains enough gravitational properties to work on a nine-qubit system and still hold true. So, then we need to ask, Are you going to learn anything about quantum gravity from the Sycamore system in this case?
I mean, a priori, you could have done the entire experiment without saying anything about AdS/CFT correspondence and linking back to wormholes. In fact, the entire quantum computation they did can also be done rather quickly on a classical machine due to the small number of qubits involved.
Balasubramanian: And in terms of there being a lab-made wormhole, it’s more like a Pixar version of a wormhole except it’s not what’s seen on screen. It’s more like the raw code running in the background; it hasn’t been converted to a decipherable image, but in theory, it could be. The image could look like a traversable wormhole, but it’s not an actual one.
Once again, they have not built a wormhole in our world. To say that they done so requires some mental gymnastics. You basically must regard the gravitational ‘dual’ description of their system as the real world.
Regardless, they have still produced a fascinating quantum phenomenon in a system that is very hard to simulate on a computer. This is a step forward in simulating complex interacting systems in quantum computers, and that’s interesting because one of the most useful applications for quantum computing is simulating physical interactions like the folding of proteins in living cells. This is incredibly hard to do on an ordinary machine, so there is an impetus to improve this technology beyond gleaning insights into theoretical physics models.
Vijay Balasubramanian is the Cathy and Marc Lasry Professor in the School of Arts & Sciences’ Department of Physics and Astronomy at the University of Pennsylvania.
Jonathan Heckman is an associate professor in Penn Arts & Sciences’ Department of Physics and Astronomy, with a secondary appointment in the Department of Mathematics.