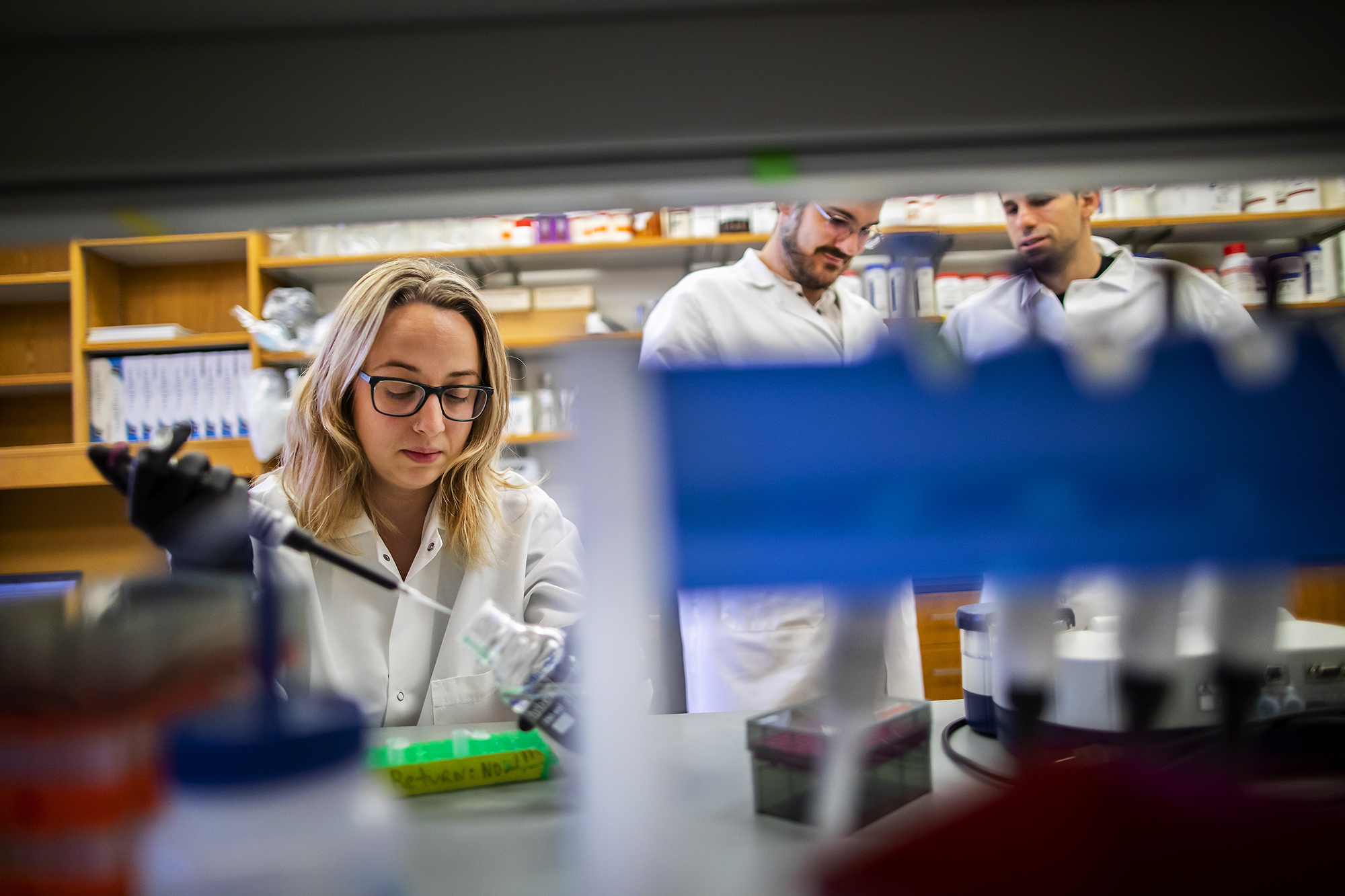
Imagine if a computer could learn from molecules found in nature and use an algorithm to generate new ones. Then imagine those molecules could get printed and tested in a lab against some of the nastiest, most dangerous bacteria out there—bacteria quickly becoming resistant to our current antibiotic options.
Or consider a bandage that can sense an infection with fewer than 100 bacterial cells present in an open wound. What if that bandage could then send a signal to your phone letting you know an infection had started and asking you to press a button to trigger the release of the treatment therapy it contained?
These ideas aren’t science fiction. They’re projects happening right now, in various stages, in the lab of Penn synthetic biologist César de la Fuente, who joined the University as a Presidential Professor in May 2019. His ultimate goal is to develop the first computer-made antibiotics. But beyond that, his lab—which includes three postdoctoral fellows, a visiting professor, and a handful of graduate students and undergrads—has many other endeavors that sit squarely at the intersection of computer science and microbiology.
Computer-generated antibiotics
Antibiotic resistance is becoming a dangerous problem, both in the United States and worldwide. According to the Centers for Disease Control and Prevention, each year in the U.S., at least 2.8 million people get infections that antibiotics can’t help, and more than 35,000 die from those infections. Around the world, common ailments like pneumonia and food-borne illness are getting harder to treat.
New antibiotics are needed, and according to de la Fuente, it’s time to look beyond the traditional approach.
“We’ve relied on nature as a source of antibiotics for many, many years. My whole hypothesis is that nature has perhaps run out of inspiration,” says de la Fuente, who has appointments in the Perelman School of Medicine and the School of Engineering and Applied Science. “We haven’t been able to discover any new scaffolds for many years. Can we digitize that information, nature’s chemistry, to be able to create and discover new molecules?”
To do that, his team turned to amino acids, the building blocks of protein molecules. The 20 that occur naturally bond in countless sequences and lengths, then fold to form different proteins. The sequencing possibilities are expansive, more than the number of stars in the universe. “We could never synthesize all of them and just see what happens,” says postdoc Marcelo Melo. “We have to combine the chemical knowledge—decades of chemistry on these tell us how they behave—with the computational side, because a computer can find patterns unlike any human could.”
Using machine learning, the researchers provide the computer with natural molecules that successfully work against bacteria. The computer learns from those examples, then generates new, artificial molecules. “We try this back and forth and hopefully we find patterns, new patterns that we can explore, instead of blindly searching,” Melo says.
The computer can then test each artificial sequence virtually, setting aside the most successful components and tossing the rest, in a form of computational natural selection. Those pieces with the highest potential get used to create new sequences, theoretically producing better and better ones each time.
De la Fuente’s team has seen some promising results already: “A lot of the molecules we’ve synthesized have worked,” he says. “The best ones worked in animal models. They were able to reduce infections in mice—which was pretty cool, given that the computer generated the whole thing.” Still, de la Fuente says the work is years away from producing anything close to a shelf-ready antibiotic.
Bluetooth Band-Aids
Beyond the computer-generated antibiotics, the research team has already created a prototype for a biosensor-lined bandage that can detect infection before symptoms occur or become noticeable to the person with the wound. Here’s how it works: Each bacterium produces unique molecules. An electrode-covered biosensor placed on an open wound can identify whether a bacterium is present, even in miniscule concentrations.
“Our sensors are detecting bacteria with even just 100 cells,” explains Marcelo Torres, another postdoc in the lab who has been closely involved with this work. “Often you need 10,000 or even 100,000 cells for something to be considered an infection. We can detect infection early and we can detect growth over time.”
Once the sensor notices an infection developing, it notifies a user via a phone-based app. With the press of a button, the user can send an electric signal to trigger release of the treatment, a bacteria-specific amino acid chain of tiny proteins called peptides pre-loaded onto the bandage. “The molecules that these bacteria produce, if you introduce a current into the system, they undergo electrochemical processes. This is very well-known,” Torres says. “What we’re trying to do is find the right patterns, the right electrochemical profile of each molecule.”
Already the team has figured out which peptides can succeed at targeting the specific pathogens causing infection and has created a working sample of the electrode and the app.
“The proof of concept is there in every step,” Melo says.
“It’s a matter of integrating it now,” Torres adds.
Once that happens, a system like this could uncover an infection much faster than happens today. Typically, results from a swabbed wound take hours or days to come back, but these readings take seconds. Future versions could be ingestible, in the form of a pill that detects certain bacteria in the gut and discharges a therapy to fight against it, de la Fuente says.
Employing probiotics
That idea—a pill that reads something in the gut then releases a treatment—is at play in another lab project focused on engineering probiotics, the “good” bacteria found in foods like yogurt.
It’s an area of interest for postdoc Esther Broset, who says that the aim isn’t to kill off the beneficial microbiome bacteria, but rather target organisms in the gut that could make us sick. “If we kill dangerous bacteria in our microbiome, what will happen?” Broset says. “The idea is to express this in probiotics.”
The same machine learning that’s narrowing down the molecules in the lab’s computer-generated antibiotic research is employed here to find the proteins appropriate for use with probiotics, crucial given that certain peptides are toxic to them.
“Say they find one peptide against a pathogen in our stomach, it causes ulcers,” Broset explains. She can then encode that protein into the DNA of specific bacteria, little by little, which allows those bacteria to produce the good probiotics. “You can code whatever you want,” she adds, “the way they’ll express, how much, in high or low amounts.” Under the right circumstances, the probiotic bacteria in the gut will produce the peptide and secrete it to counter the pathogen.
Right now, one of the most promising targets is Salmonella, de la Fuente says. “In theory, you could take yogurt, it would go through the body, reach your colon, your gut, and the probiotics would colonize the gut and treat the Salmonella infection.” Eventually, if the concept proves successful, the conduit for the probiotics could be a pill taken proactively that releases the appropriate peptide when it detects dangerous bacteria.
Ultimately, for all of these research projects, the goal is to create what de la Fuente describes as a closed loop of discovery, where everything from computational design and machine learning to printing, synthesizing, and screening for biologicals gets automated and looped back to the computer. “For molecular discovery and creating new medicines, it can be an incredibly useful set of tools,” de la Fuente says.
He’s also made a commitment to create a diverse lab with people from groups often underrepresented in these fields and who come from a range of places and scientific backgrounds. “The lab’s ethos is to welcome talent from around the world, and to empower these individuals to become the leaders of tomorrow,” he says, adding that this mix of scientists allows the lab to push the boundaries in molecular sequencing.
“We’re trying to explore previously unexplored regions that may yield molecules that we’ve never seen before because they don’t exist in nature,” he says. “I’ve always been interested in trying to learn the secrets of diseases and processes.” Taking a page from the playbooks of computer science, AI, synthetic biology, microbiology, and many other fields, the team is getting that much closer to answers that could have a lasting impact on health worldwide.
César de la Fuente is a presidential assistant professor and leader of the Machine Biology Group. He has appointments in the Perelman School of Medicine and the School of Engineering and Applied Science at the University of Pennsylvania.
Marcelo Melo, Marcelo Torres, and Esther Broset are postdoctoral research associates in the de la Fuente lab in the Perelman School of Medicine and the School of Engineering and Applied Science at the University of Pennsylvania.
Homepage photo: César de la Fuente has always been fascinated by biology and the world around him, how we communicate, how animals behave, how cells function. During his training, he noticed there wasn't a precise, predictable way to program proteins. “I realized one of the solutions would be to incorporate concepts from computer science and synthetic biology, to truly program these molecules well, as opposed to seeing what happens when they mutate.”