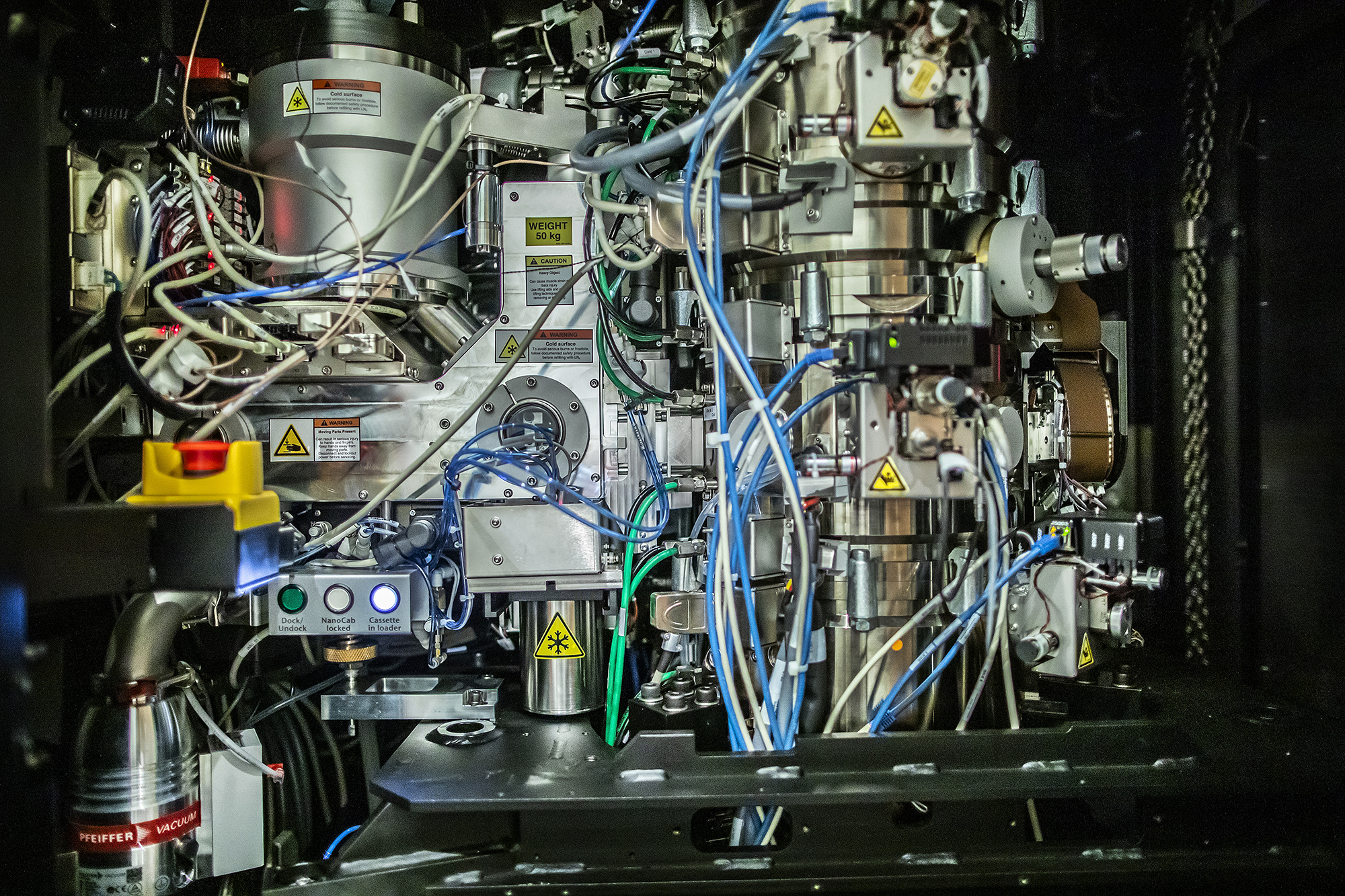
Penn’s Singh Center for Nanotechnology has added the newest cutting-edge technology to its collection of already impressive microscopes: The Krios G3i, a cryogenic electron microscope that will allow researchers to look at cells, proteins, and engineered nanoparticles like never before. The trick is to keep them frozen in time, holding them in their natural environment while bombarding them with ultrafast, ultrahot subatomic particles.
“Cryo-electron microscopy, or cryo-EM, will allow scientists at Penn to understand diseases like cancer, Alzheimer’s, Parkinson’s, and heart and kidney disorders. Biomedical engineers will use cryo-EM to improve nanoparticle technology for drug delivery. Bringing this microscope onto campus is a big deal for everyone,” says Vera Moiseenkova-Bell, associate professor of pharmacology in the Perelman School of Medicine and faculty director of the new Beckman Center for Cryo-Electron Microscopy.
The Arnold and Mabel Beckman Foundation, together with the School of Medicine made this Nobel-winning technology available on Penn’s campus last year, allowing scientists to look at molecules in their natural state at a nearly atomic level.
The ‘cryo revolution’
Moiseenkova-Bell is already seeing firsthand the advantages of using cryo-EM.
“Getting the structures of biologically important molecules at an atomic resolution is now possible because of the cryo revolution. Compared to other techniques, this is so fast. Crystallography can take sometimes over a year to get one structure. Cryo-EM allows you to get a structure in days.”
Many structural biologists like Moiseenkova-Bell consider cryo-EM to be a revolution in their field because of the ways it both supersedes and complements other imaging methods currently available, namely nuclear magnetic resonance (NMR) and X-ray crystallography. Cryo-EM fills the hole left by NMR and X-ray crystallography: Not only can it image the larger molecules excluded from NMR, but it skips the demanding crystallization process of X-ray crystallography.
“With NMR we can only get a small number of protein structures due to the size limitation, and with crystallography you need a well-ordered crystal to get only one structure [of the molecule]. With cryo-EM, you could visualize pretty much everything that is bigger than hemoglobin without needing to crystalize and solve many structures at a time,” Moiseenkova-Bell says.
An added benefit of cryo-EM is that it allows scientists to look at structures in their natural state without crystallizing or dehydrating them. While crystallization requires scientists to take proteins and unnaturally force their atoms into a perfect crystal lattice before imaging, cryo-EM presses pause on a cell’s natural processes, allowing researchers to look at where proteins are and more accurately infer how they work.
“Because the proteins get flash frozen in liquid ethane, we are able to trap them in their natural conformations. That’s another benefit of cryo: The proteins, or cells, are in their native environment which is impossible in other techniques,” says Moiseenkova-Bell.
How to freeze time
The “cryo” and the “EM” portions of the microscope are actually separate processes. What allows cryo-EM to capture a sample in its native state is the “cryo” freezing that takes place when researchers are preparing a sample. Samples are plunged into liquid ethane at temperatures below -315°F, seven times colder than the average temperature of the North Pole during winter, instantly freezing them solid. This process is known as vitrification: Unlike normal freezing, which might form ice crystals that could hinder the imaging, the liquid ethane fixes the sample in a glass-like block that preserves its structure.
The transmission electron microscope, the “EM” part of cryo-EM, works by sending a beam of energetic electrons through the sample. In other EM imaging methods, the powerful beam of subatomic particles can destroy the sample, but cryo-EM’s vitrification process, along with its low electron dose imaging, protects the sample from damage. The electrons’ interactions with the sample’s atoms are detected by the direct electron camera and used to reconstruct a 3D image of the sample.
This last step is crucial: The detectors and the computer program that collect and create images were the final parts of the cryo-EM technique to fall into place. The individual “cryo” and “EM” technologies have been around for decades, metaphorically collecting dust as some structural biologists dismissed the cryo-EM method entirely. Others, however, waited hopefully for the time when technological conditions would be ripe for the cryo-EM revolution to take off.
“The modern transmission electron microscopes are very advanced and can produce high-resolution images. It’s the development of the medium that we collect images of the biological samples on and the software to reconstruct 3D structures from these images that revolutionized everything. It was a technological evolution,” says Moiseenkova-Bell.
Prior to these advances, cryo-EM relied on photographic film or charge-coupled device cameras to capture images of molecules and cells. On top of the challenges presented by low-quality film and low electron dose imaging, frozen cells continue to move ever so slightly during imaging. Combined, these factors resulted in blurry, often indecipherable photographs of molecular structures, earning cryo-EM the nickname “blob-ology” among structural biologists.
Now, updated technology allows scientists to capture mini-movies of proteins and remove any motion blur from the images. These crisp images of molecules analyzed by powerful computational methods allow scientists to see protein structures at atomic or near atomic resolution.
Looking ahead
With its newly elevated level of resolution, Moiseenkova-Bell sees cryo-EM as a tool that can benefit Penn researchers across disciplines. On the engineering side, cryo-EM offers scientists the chance to more precisely engineer nanoparticles that require each atom to be placed with purpose. On the medical side, researchers hope to use cryo-EM to better understand protein structure and function and in turn, how to best design effective drugs to treat a variety of conditions—a use that is also attracting the attention of big pharmacological companies in Philadelphia.
“Membrane proteins represent 60 percent of druggable targets. If we can know the structure of each target at atomic resolution, we could understand their function and then start doing novel structure-based drug development for these targets,” says Moiseenkova-Bell. “Or, we could solve the structures of proteins with known therapeutic compounds and see how we can improve their effect. The options are endless for drug discovery.”
Part of the allure of Penn’s cryo-EM is its accessibility. Not only will Penn researchers of many disciplines have access to its capabilities, but the machine could attract collaboration with researchers and companies all over the northeastern United States. The Singh Center is already part of the National Nanotechnology Coordinated Infrastructure, which aims to provide access to specialized resources for nanotechnology researchers, and the addition of cryo-EM expands the resources that Penn can offer to valuable research endeavors.
Eric Stach, professor in the School of Engineering and Appled Science and chair of the Faculty Oversight Committee at the Nanoscale Characterization Facility, sees Penn’s cryo-EM as teeming with potential for insightful research and collaboration.
“The co-location of this forefront instrument in the Singh Center for Nanotechnology will provide unique opportunities at the interface between biological sciences and nanotechnology,” he says. “[Additionally,] Penn recently won a National Science Foundation Major Research Instrumentation grant to install a unique electron microscopy sample preparation instrument—a Cryo Focused Ion Beam Microscope.”
The Cryo Focused Ion Beam Microscope accelerates ions into a sample, rather than using electrons like EM devices. Although the instrument can be used for imaging, the microscope is also useful as a cryo-EM preparation device because, at its highest energy levels, the laser-like beam of ions can cut frozen samples into thin slices that are suitable for subsequent imaging in the Krios G3i machine.
“[Having access to this instrument] will allow researchers to use the cryo-EM to effectively sample the interface between ‘soft’ biological structures and ‘hard’ materials,” says Stach. “This will enable entirely new areas of scientific exploration across multiple research fields.”
Support for Penn’s cryo-EM facilities and equipment comes from the Arnold and Mabel Beckman Foundation and the National Science Foundation through grant DMR-1828545.
Homepage image: Cryo-Electron Microscopy Core Director Darrah Johnson-McDaniel loads a sample into the Krios G3i, a technology made available to Penn’s campus thanks to the Arnold and Mabel Beckman Foundation and the Perelman School of Medicine. (Image: Eric Sucar)
This story is co-written by Lauren Salig and Erica K. Brockmeier.