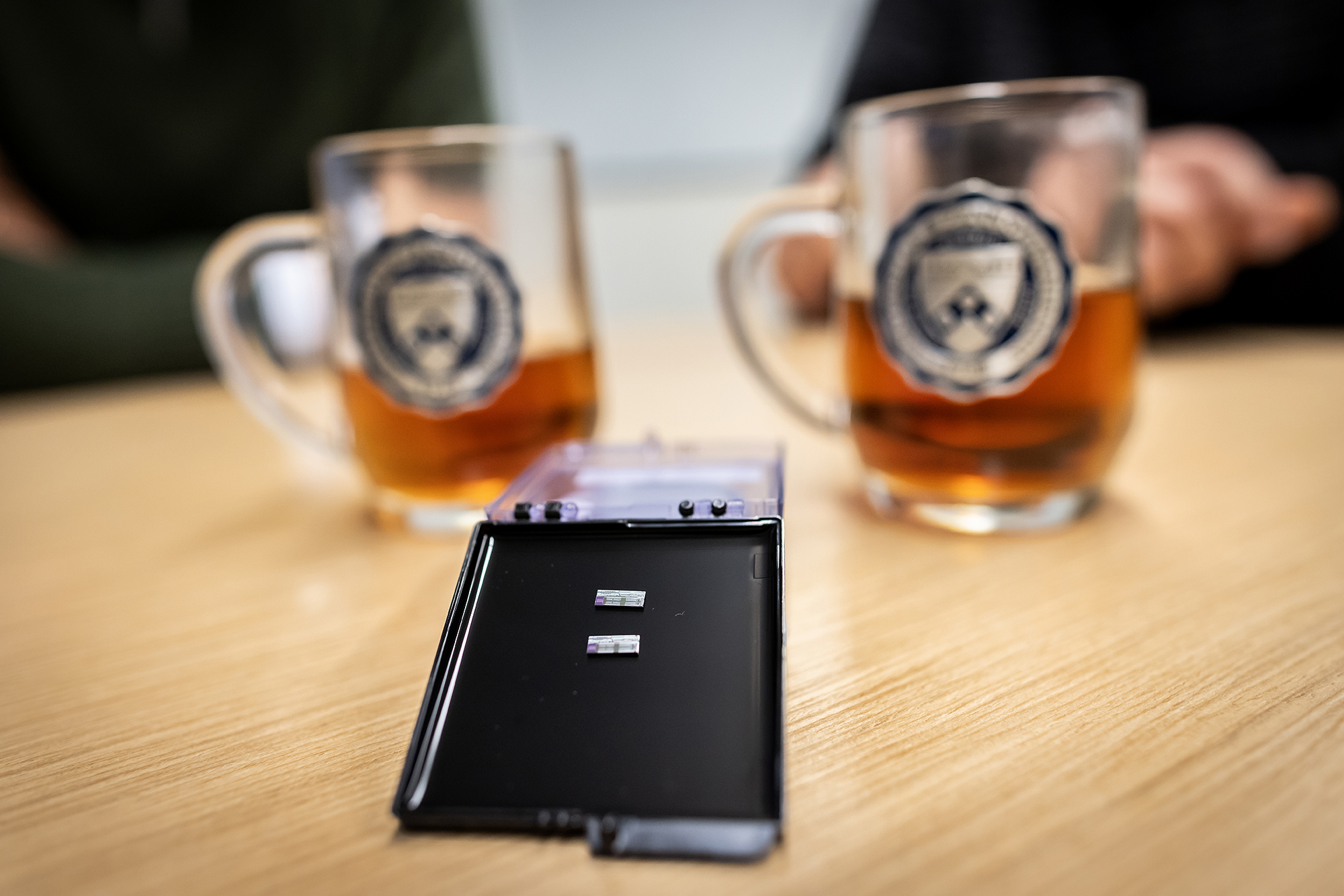
According to Chinese legend, the first cup of tea was an accident. Shennong, a mythical emperor, boiled a pot of water, only for the wind to add a handful of leaves.
In Penn Engineering’s Department of Electrical and Systems Engineering (ESE), tea leaves likewise result in happy accidents.
Nader Engheta, H. Nedwill Ramsey Professor, regularly joins his colleague Firooz Aflatouni, associate professor and undergraduate chair in ESE, for a cup of tea in the latter’s office. “We talk about academic life,” says Engheta. “We talk about history, politics.” And, of course, science.
Engheta, who won the Benjamin Franklin Medal last year, is known for his groundbreaking contributions to the design of materials that interact with electromagnetic waves at tiny scales with unprecedented functionalities. More than a decade ago, the Department recruited Aflatouni, who specializes in the design of electronic and photonic chips, and Engheta became his mentor. “We come from different angles to the field of optics,” says Engheta.
Over tea, the two brew up new ideas. While perhaps not as directly inspired by teatime as James Watt, who famously experimented with kettles en route to inventing the steam engine, the pair nonetheless finds that ideas rise like the steam from their teacups. “It’s a pleasure to collaborate with Firooz,” says Engheta. “We love to see how we can bring our ideas together.”
How tea catalyzes collaboration and recent results
Most recently, the two devised a novel chip that uses light rather than electricity to perform vector-matrix multiplication, a key mathematical operation in the training of neural networks, the computer systems powering AI tools like ChatGPT.
“The potential impact is enormous,” says Stefano Maci, professor of electromagnetics and antennas at the University of Siena, and a longtime professional colleague of Engheta’s, “since matrix multiplication is fundamental to many operations in AI and machine learning.”
Inspired by biological brains, neural networks store information as long lists of numbers, known as vectors. Each time the network learns something new, the numbers must change, just as the synapses connecting neurons strengthen or weaken in response to new experiences.
To encode new knowledge, the network multiplies each vector’s initial values by a set of other numbers called weights, which are formatted in grids of rows and columns, an arrangement known as a matrix.
Just like the brain, which demands roughly one-fifth of the average human’s resting metabolism, the sheer number of matrix multiplications required to train neural networks consumes vast amounts of energy.
Earlier this year, the International Energy Agency reported that, by 2026, data centers worldwide—where companies like Google, OpenAI, and Meta develop neural networks—will require as much energy as Japan.
Conventional computer chips require so much energy in part because of the nature of electricity. Unlike lightwaves, which can travel in parallel without disrupting one another, the electrons moving through traditional computer chips have to achieve one function after the other. Computer chips even have a “clock” signal to synchronize the passage of information.
In a chip powered by light, by contrast, everything happens almost at once, at the speed of light. “As the lightwave travels, the computation takes place,” says Aflatouni.
In the time it takes a traditional computer chip to perform a single matrix multiplication, a light-based chip can perform all required multiplications within the network and classify the input data.
Similar paths, different waves
In a curious way, the careers of Engheta and Aflatouni echo one another. Both researchers immigrated to the United States from Iran, after earning bachelor’s degrees from universities in Tehran. They each then earned doctoral degrees in Los Angeles: Engheta studied at the California Institute of Technology (Caltech) and Aflatouni at the University of Southern California (USC) and then Caltech for his postdoc.
Both then made their way to Penn Engineering, Engheta in the 1980s and Aflatouni the 2010s. Their connection started simply because of their shared interest in light, but strengthened into a bond. “Nader is my mentor,” says Aflatouni.
“To have Nader as a mentor, as a colleague, as a supporter — I can't imagine a better blessing for a younger professor,” says Alan Willner, one of Engheta’s longtime professional colleagues and the Andrew and Erna Viterbi Professorial Chair and Distinguished Professor of Electrical and Computer Engineering at USC. “There’s only one Nader.”
As Willner sees it, collaboration is one of Engheta’s superpowers. “Nader is like Lebron,” says Willner, not just because, like Lebron James, Engheta is known by his first name to virtually everyone in the field, but because “they up everybody else’s game.”
For Willner, this is what sets Engheta apart as a collaborator. “His star, no matter how bright it shines, never diminishes and overshadows other people. He just enhances everybody around him with encouragement, with great ideas, with mentorship, with warmth.”
Perhaps unsurprisingly, Engheta and Aflatouni approach their research from complementary directions. “We always start from the theory,” Engheta says of his group. Aflatouni and his group, by contrast, focus on the experimental aspects—fabricating and measuring, rather than theorizing, new chips.
“A lot of times we get together,” says Aflatouni, of his and Engheta’s teatimes, “basically talking about the really interesting ideas coming from Nader and his group, and have a back-and-forth of me saying, ‘Oh, this, we can do this. We cannot do that.’”
The two wondered if it would be possible to apply the theory behind one of Engheta’s earlier projects—in which he developed a material that could compute and solve integrals equations, another important mathematical operation—onto a smaller platform, made entirely of silicon, using lightwaves rather than microwaves. “That’s where the expertise of Firooz came into play,” says Engheta.
Fabrication challenges
The number of commercial foundries or “fabs” capable of producing chips like the one Engheta and Aflatouni imagined is exceedingly small, and the pair wondered if a foundry would even accept their new design.
Foundries typically only accept submissions a few times a year, through a process known as the “tape out,” a reference to when chip designs came on magnetic tape, and have strict rules about what can and cannot be fabricated. “Maybe the radius of curvature of one part of the chip turns out to be two nanometers,” says Engheta. “The foundry might say, we cannot make two nanometers—go back and redesign it.”
As a result, Aflatouni and Engheta had to repeatedly redesign the chip, essentially making it conform to commercial specifications and eligible for mass production.
Fortunately, Engheta and Aflatouni weren’t alone in attempting to overcome this hurdle—they had extremely capable collaborators, who brought their own mugs to the conversation.
More mugs
Prior to joining the Engheta Group, Vahid Nikkhah, a doctoral candidate and the first author of the latest paper, was also an avid tea drinker. “But since leaving Iran and moving to the U.S.,” he quips, “I’ve mostly switched to coffee.”
Much like Aflatouni and Engheta, Nikkhah, who has a background in physics and electrical engineering, has long been enthusiastic about imagining how to use waves to do more than stir about flavonoids in a teacup.
“I was really drawn in by Professor Engheta’s approach to tackling the fundamental goals of physicists working in this space,” Nikkhah says. “Using light waves for computation isn’t wholly new, in the sense that researchers have been exploring this since the 1960s, but altering the very nature of materials at the atomic scale to sculpt the path of light and perform calculations presents an exciting, boundless paradigm in computing.”
This process, Nikkhah explains, is basically like channeling light through a tunnel, where a series of strategically placed objects in the middle distort and bounce the light, so that by the time the light reaches the other side, it has been measurably transformed.
The light from the source represents an input for this system; the altered light at the end of the tunnel is the output, now encoding new information that depends on how the light was manipulated.
These transformations, of course, have to be both desirable and predictable—two extreme challenges when working with materials at tiny scales. The objects that interact with the light are known as “metamaterials,” a special class of human-made materials whose elements are assembled in ways that imbue them with properties rarely found in nature.
In contrast to Nader and Aflatouni’s focus on the overall design of the new chip, Nikkhah worked on structuring the metamaterials themselves so that they altered some of the incoming light’s fundamental properties, such as the height of its waves (amplitude), position in its cycle at a given time (phase), orientation of its oscillations (polarization), the distance between successive wave crests (wavelength), and the number of wave crests passing a point per second (frequency), in as predictable manner as possible.
The result, as Maci at the University of Siena, describes it, is a silicon microstructure with input and output ports.
The whole process is performed at the speed of light, and the entire structure takes up a minuscule amount of space: The flat, silicon chip is just about 23 microns (or millionths of a meter) in width and about 34 microns in length. For context, one micron is about 1/100 the thickness of a strand of human hair.
To engineer the metamaterials so that they could consistently manipulate these properties of light, Nikkhah employed inverse design, a method by which the desired outcomes are specified first, and then the structures necessary to achieve these results are uncovered using optimization algorithms to determine the best approach.
“Inverse design may seem unintuitive,” says Nikkhah, “but that’s where one of its strengths lies. It often involves numerical and topology optimization, where the material layout within a given design space is iteratively adjusted to make sure it’s consistent with the calculated outcome. This is what gives us configurations that go beyond what we as designers can achieve by guessing what would work.”
To achieve the team’s objective—making a chip that could perform vector-matrix multiplication using light—Nikkhah designed “low-index contrast” structures.
In this context, contrast refers to the difference in refractive indexes between the various regions of the metamaterials—with a low contrast indicating a smaller difference—which helps minimize the unwanted reflections and light scattering and leads to more efficient and predictable behavior as the light travels through the material.
“This method also lets us model light’s behavior in a more computationally efficient way when compared to traditional three-dimensional simulations,” Nikkhah adds.
Willner, at USC, finds the team’s approach to simplifying the design process particularly innovative. “Inverse design,” he says, “enabled them to have an incredibly efficient process and to achieve excellent performance.” He also believes that their findings presage a more efficient, elegant means of transmitting information well beyond the chip’s potential application in AI.
“There’s really very little power loss due to heat,” he notes Willner, since light encounters little resistance as it moves through the chip. Electrons, by contrast, throw off heat as they encounter resistance when traveling through conventional chips, which is why the fan on your computer turns on, and why today’s data centers need massive cooling systems.
Willner says data centers using light-based chips would use much less energy, leaving a smaller carbon footprint. What’s more, using light instead of electricity means more information can be carried at once. “The higher the frequency, the more data capacity you can handle,” says Willner. “The smaller the wavelength is, the more you can do in a smaller footprint.”
Andrea Alù, a former postdoctoral researcher in Engheta’s lab who went on to found the Photonics Initiative at the City University of New York, where he is also a distinguished professor and the Einstein Professor of Physics, likewise foresees chips like this one playing an integral role in future computers.
“The possibility of performing canonical operations, such as matrix multiplication, on a chip using light is very compelling,” says Alù, “as it would drastically reduce the energy needs and timescale of computations commonly done today with digital computers.”
Reading the tea leaves
Just like a good tea time conversation, innovations like Engheta and Aflatouni’s new chip testifies to the value of collaboration. “The success of ideas like the one presented in this paper,” says Maci, “often stems from the collaborative efforts of researchers who bring together their diverse expertise, perspectives and resources to tackle complex challenges and drive innovation in their respective fields.”
Nikkhah says turning the group’s idea of inverse design into a reality hinged on bringing together people with different skill sets. “While I focused on the theoretical and numerical optimization side,” he says, “others had expertise in fabricating and testing the devices."
Looking ahead, Nikkhah says that one of the most exciting prospects of inverse design is the ability to render structures reconfigurable. In other words, future light-based chips could have materials that change properties with external excitation, allowing for dynamic control of optical devices.
At the moment, optical computing as a subset of analog computing is faster and more energy-efficient than traditional, or digital, computing. But whereas digital computers are reprogrammable, meaning that a single chip can be rejiggered to perform many different tasks, analog devices, like Engheta and Aflatouni’s new chip, can only perform the function for which they were designed—in this case, vector-matrix multiplication.
“In a conventional computer, you have one system, but you can do many things with it,” Engheta explains. “In our system, the chip does this function of multiplication. But imagine that you could also change the patterned material. That would mean you could change the matrix, and that would mean the chip could be reprogrammable in the future. We’re not there yet, but that would be one of the directions for a follow-up.”
If the team manages to pull that off—creating a chip that can perform calculations at the speed of light, using barely any energy, and be reprogrammable—the result will be brewed from more collaborative conversations. “It’s one of those situations where the result is larger than sum of the parts,” says Engheta, of the new chip.
Or, as Aflatouni puts it, “sometimes, one plus one really is more than two.”
Ian Scheffler of Penn Engineering contributed to this story.